Abstract
The goal of this study is to implement the Developmental Instruction approach in the middle school science curriculum. We assume that ancient technologies can serve as a proper cultural-historical context for creating meaningful classroom activities such as experimenting and model building. This context is realized by means of a series of short texts that describe ancient technologies and let students interpret them in terms of the already learned material. The text-lab-model triad as a unit of model building and development is discussed. We have realized these principles in two linked science courses for students from 5th to 7th grades, called “Narture” and “Introduction to Chemistry”; the latter can serve as a prelude to the systematic chemistry course starting at 8th grade. The features of the texts used in these courses, the organization of “students” experimental work, examples of model building, and students’ outcomes are presented. The approach promotes students’ critical thinking, conceptual understanding, learning initiative, and learning independence.
Keywords: Developmental InstructionEducational Design ResearchLearning InitiativeCultural-Historical Approach
Introduction
Teachers and educators consider 10-12-year-olds, or tweens, as very sensitive to social and cultural norms; this is the age where many habits affecting the subsequent major life decisions are formed. When it comes to education, children discover that the norms and goals valued in their community and society differ significantly from what they are taught at school, and this disconnect is acknowledged as a source of a great social conflict in 15-17-year-olds (Zuckerman, 2007). Well-known issues among the tweens include a significant loss of interest in education, development of various health problems, a rise in risky, reckless, or deviated behavior, and among them a widespread decline of the intention to continue education.
We believe that modernizing the organization of teaching and learning might be crucial to combat this trend. This approach dates back to 1958 when D.B. Elkonin and V.V. Davydov initiated a long-term project aimed at promoting students’ critical thinking and learning independence starting from elementary school. However, it is not clear yet how these ideas can be implemented in middle school.
The long-term goal of our study is to expand the approach by Elkonin and Davydov’s to middle school. Our particular focus is on the subject areas of natural sciences. We are trying to design a curriculum and teaching strategies appropriate for tweens and assess its developmental potential.
Problem Statement
Any attempt to implement the Developmental Instruction approach into the middle school brings about a lot of problems because the entire curriculum has to be reworked, and ideally, this should be done for all subject areas. The ultimate goal of the project is to rethink the middle school standards and change the regular practice. We focus here on the subject domain of natural sciences: the basics of physics, biology, physical geography, and especially chemistry.
The Developmental Instruction approach to Teaching Science
Here are the main features of the approach as we implement it in the middle school science course:
The basic concepts of natural science have originated from the human activity, with the primary goal of converting various natural objects into useful things and tools and using natural phenomena for humans’ sake. The concepts are mental tools that help to plan how to reach the goal and can predict the result of the action chosen (cf. Taber, 2010). To be applied adequately the concepts have to be learned as planning and predicting tools.
Concepts are derivatives of certain actions. To learn a concept adequately, students have to master the related actions. Some examples from the earliest stages of the course are presented in Table
01 .Basic concepts form a stratal structure where different models occupy different levels, each working in its own context (cf. De Vos & Pilot, 2001; Taber, 2010). The students should be familiar with this construct.
Early stages of model building should be organized as joint actions to make sure that the students grasp the crucial components of the model. A partner as an opponent helps to realize why certain actions are essential for the success (Rubtsov, & Martin, 1991). Anything complicated should be built first in the students’ actions in the process of collaboration; this is a necessary step for a future interiorization of the concept.
Historical Materials in Teaching Chemistry in Middle School
Researchers have made many attempts to incorporate historical materials into the teaching of systematic science courses and chemistry in particular (Matthews, 2014). Various approaches have been put forward, but the majority of them relate to high school students or undergraduates. For younger students educators (Scheffel et al, 2009) suggest focusing on the similarity between the historical models and the students’ misconceptions regarding science concepts. The limitations of the historical models are considered as a good starting point for a discussion about inconsistencies of student’s ideas about the natural world, their misconceptions, and discrepancies between different parts of the models that students may have.
By contrast, we prefer to emphasize the positive sides of the historical models, especially those that still work inside and alongside the modern models and can serve as stepping stones towards them. We strive to build the necessary models with students, such that the students can learn their internals in order to be able to identify an appropriate context where each model can be used. Rather than being a mere opportunity to start the conversation, the historical materials are put in the center of our approach.
Research Questions
-
What are core concepts that can serve as a solid basis for an introductory science course for middle schoolers? What essential models do they present? What activities make students grasp the concepts as planning and predicting tools? We assume that the subject-specific concepts in question are force in physics, molecule, chemical reaction, and chemical element in chemistry, living cells in biology, etc. At first, we designed and tested an introductory chemistry course for 6-7 graders (Vysotskaya, Khrebtova, & Rekhtman, 2015). Then, the same approach was applied to certain related scientific concepts, and a new version of our science course for 5-graders is currently being tested (Vysotskaya, Khrebtova, Lobanova, Rekhtman, & Yanishevskaya, 2018).
-
What action should students learn to get a particular concept as its derivative? How have the actions and the related models and concepts developed into their modern forms? What did their primary forms look like? What are the necessary historical steps of such development that have to be presented to the students, and what steps can be skipped? Does withholding certain developmental steps affect students’ understanding and/or ability to adequately apply the concept?
-
How can the primary form of action be presented to students? How can such an action be organized as a joint action? How should it be developed further toward its mental form?
-
What cultural-historical context can support the early stages of model building and make the activity meaningful for students? How can such a context be presented to the students? We assume that the ancient technologies that people used in the past for their various needs can be the key part of the answer.
Purpose of the Study
The main purpose of our long-term ongoing study is to improve the science teaching at school through the implementation of the Development Instruction approach that fosters students’ learning independence and promotes students to be in charge of their own learning (Zuckerman, 2010). Unfortunately, after regular science classes, the majority of students do not understand the basic science models, cannot solve problems that simply reformulate the standard problems, and cannot apply the learned concepts in real-life situations. Moreover, many students consider regular science classes either too hard or too boring and do not choose advanced science electives. So, our intention is to design introductory and systematic science courses (such as chemistry, physics, biology, physical geography) that make students understand natural sciences, their role in human history and in the modern society, appreciate their findings and methods, and be ready to take advanced science courses as electives.
Other goals include testing our assumptions and hypotheses about how the Developmental Instruction approach can be implemented into middle school, testing our local instruction theories regarding certain subject areas and particular concepts, and testing certain hypotheses about the mechanics of concept learning.
Research Methods
For our teaching experiments, we use the Educational Design Research Framework (cf. van den Akker, Gravemeijer, McKenney, & Nieveen, 2006; Plomp, & Nieveen, 2013). The first introductory pilot course in chemistry was taught in 1995/96. Since that time, it has been taught every year, and we have revised and refined it several times. Currently it can be taught in three different modifications: as a 2-year course for 6-7 graders following our science course, as a standalone 2-year course, and as a short 1-year course for 7-graders.
We closely monitor the teaching and learning processes in our experimental classrooms. We teach, listen to, and support our teachers and teachers-to-be; monitor students’ attitude, progress, and outcomes during the experimental macro-cycle and beyond it, i.e., in the subsequent grades (8-11) in high school. We are constantly adjusting our teaching materials according to the teachers’ and students’ feedback, as well as our own findings and analysis.
Designing every new teaching module requires an analysis of the logic and history of all related concepts and technologies, including the cultural context of their origin and development. Such genetic analysis uncovers a specific stratal structure of a concept and the history of its modifications. As a result, we can choose the historical models that are essential in enabling the students to grasp the concept as a planning and predicting tool. Besides, we can determine what cultural contexts can be helpful to make the related technologies meaningful to students, and so on.
Findings
Here is what we found while implementing the Development Instruction approach to teaching science in middle school.
The Basis of Science
Natural sciences stem from human purposeful activity to make necessary and useful things from natural objects and materials; observations of natural phenomena are not sufficient for it (cf. Meijer, Bulte & Pilot, 2009; Talanquer, 2013; van Aalsvoort, 2004; van Berkel, Pilot & Bulte 2009). So, to make science understandable for students from the very beginning, this idea should be at the center of the teaching strategy. There is one activity that has shaped chemistry and its concepts; this activity is preparation of a certain substance from naturally available substances. At first, substances were described by their properties, and only when the modern chemistry was born, by their composition. So as a prerequisite, the students have to be familiar with the notion of “substance” and “chemical reaction” and master at least the two first items of the Basic Particle Model (Vysotskaya et al., 2015). For us, this was the main reason to focus on how these notions should to be introduced to younger kids, such that the subsequent learning of chemistry is given the proper basis. All substances that the mankind learned in the past served certain people’s needs, and for this reason, the processes of preparation of these substances were of interest. This cultural-historical context should be included into the introductory course to let students know why they learn about certain substances and processes, and what roles these substances and processes played in the past (served people’s everyday needs) and in the history of science.
The Basic Model for Chemistry: “Introduction to Chemistry” for 6-7 graders
We have found that among the primary chemical concepts, chemical element is the most fundamental in the sense that it gives rise to all others. Its first layer is a notion of something that is conserved in chemical reactions while substances change. The historical context of this construct is also very important. In order for chemistry to become understandable, the students should discover, test, and make sense of it all. It implies that significant time should be devoted to building of models with the students; in so doing, development of the model of chemical reaction opens a venue to the idea of substance composition. This should also include all related actions that allow students to understand what is going on. Thus, the primary model that students build and develop further is the element cycle (Vysotskaya et al., 2015). Table
A Unit of Model Building: Text-Lab-Model Triad
We have found that a text-lab-model triad can serve well as a unit of model building with students. It works as follows:
Students read a short text that describes an ancient technology and interprets it in terms of the already learned material.
Students build a model and test it by planning simple experiments that would reproduce the important steps of the technology on their lab tables. Subsequently, they carry out the experiments.
Students compare the results with what the model has been predicting. All inconsistencies and discrepancies are discussed, new questions arise, and then a new text is offered or new experiments are planned.
The aforementioned texts have been written with three big no’s in mind: no explanations, no modern names and/or chemical formulas of substances, and no chemical equations. Each text describes an ancient technology using certain means of modeling that students can use to interpret it (see below). The technologies chosen serve various human needs – from baking bread to smelting metal, see Table
The simple experiments that students plan and carry out help them to test (1) whether and how the technology works and (2) whether the model they have built based on the text is correct or has to be revised. Students work with reagents that have no modern names or chemical formulas on the jars or flasks; they may be labeled either by their archaic names (the same that are used in the texts) or by the names that the students have given them previously; the latter is referred to as the parallel language (Vysotskaya et al., 2015).
At first, the models that are built with the students may have a very simple form. Subsequently, they have to be developed and differentiated, and every new step requires a whole learning cycle from reading a text to planning experiments and drawing the conclusions (see below).
Cultural-Historical Context: Ancient Technologies for Model Building
According to our experience (Vysotskaya, Khrebtova, & Rekhtman, 2016), ancient technologies make an excellent context for students’ modelling activities because of the following:
Each archaic technology has its modern descendant that serves a major human need, and it makes clear to students why people used it in the past, why people do it today, and why the internals of the technology is still interesting to modern people.
An archaic technology is based on the simplest model of the phenomena, and this makes it the best starting point for students to build a model of their own. Every next generation of the technology is based on a more complicated model that involves more factors and has to be built from the simplest model to be completely understandable and applicable by students.
The simplest model forms the first and most fundamental layer of a concept stratal structure (cf. De Vos et al.). It is essential for students to uncover and realize what it is before they learn about the subsequent, more advanced layers if we want students to apply the concept freely in all necessary situations, and especially in real life.
An overview of the course content is present below.
The Nature Works for Humans: Why & How?
Our science course for 5-graders aims at introducing them to the activities and ideas that many centuries ago gave birth to the natural sciences as we know them today. The models that students should build based on the descriptive texts and the associated experiments form the foundation of their perception of natural sciences. Teachers consider such models so trivial that usually don’t even bother teaching them because they believe that students can learn on the go. However, our findings indicate that these ideas should be taught to the students if we want the more advanced models be built on this foundation. This is a goal of our introductory science course called “Narture” (Vysotskaya et al., 2018).
We formulate the main idea of the first module as follows: “Humans’ way to survive is to make tools”. Students should realize here that every tool, even as simple as stone age hammer and axe, has its own function, and it determines how the tool looks, how it works, and from which material it is made. During this work, the students learn to ask a few important questions, such as “For what purpose was it made?” and “How did it work?”, and realize that the function of a certain tool is fundamental for finding the answers.
The next few modules are devoted to the following:
to build and comprehend the flowchart model of a technology as the first step to understand it (Fig. 01);
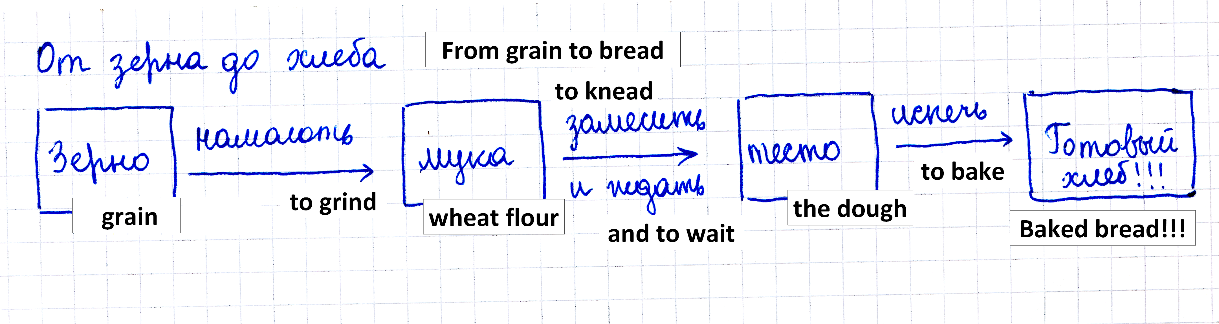
-
to start building the Basic Particle Model (Vysotskaya et al., 2015) in order to plan and predict the processes of production of various substances of interest (salt, sugar, oil etc.) – see Fig.
02 . On this basis, the students can construct the simplest model of chemical reaction, namely, that the particles of products differ from the particles of reagents.
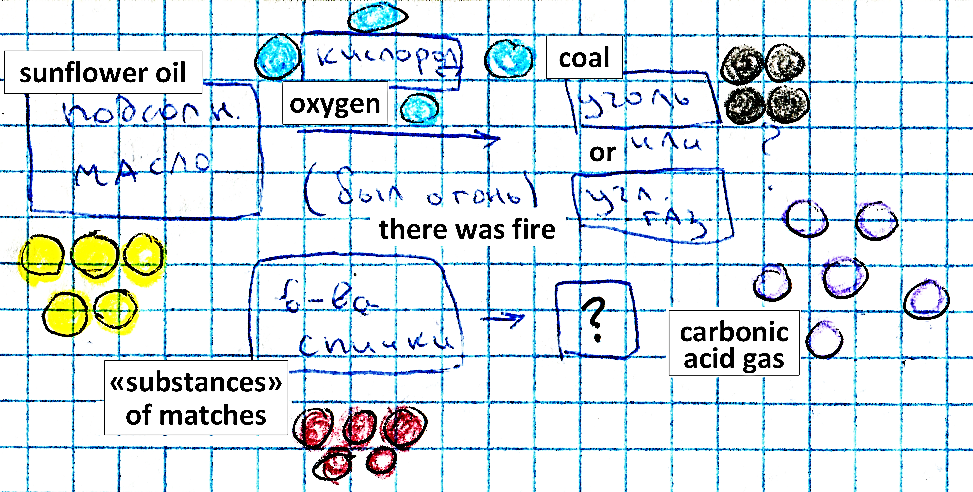
Model Building & Development
What features of the course can support students’ activity of model building? Let’s take a closer look with the model of chemical reaction as an example. Here we consider the process of smelting iron.
In our science course, the 5-graders read a text about smelting iron for the purpose of eventually making tools and weapons. They draw a diagram showing how the furnace was loaded at the start of the process (Fig. 03). Then they build a multi-particle diagram of the process based on the text (Fig. 04).
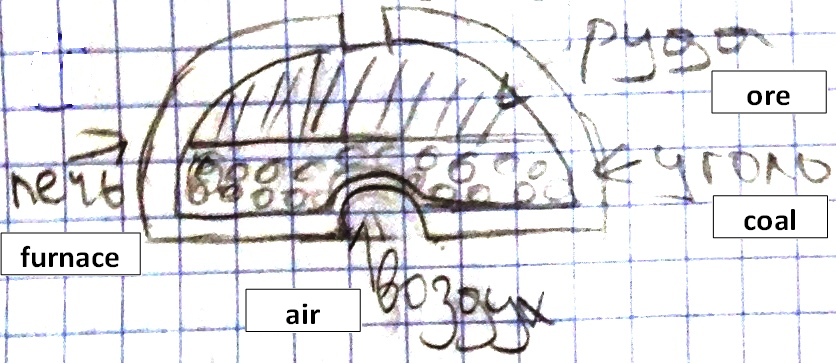
![[Iron smelting (particle model), drawn by a 5th-grader]](https://www.europeanproceedings.com/files/data/article/93/3768/ICPE2018F086.fig.004.jpg)
This is the simplest possible model of the reaction of interest: it only reflects the fact that every substance named in the text has different particles (ore, coal, iron), but it does not discuss other products of the reaction because this question simply cannot appear at this level. However, the idea that the product of reaction is different from its reagents is emphasized here.
In the course called “Introduction to Chemistry”, the 6-graders study iron, rust, and their properties and transformations by planning and carrying out simple experiments. Based on the results, the students can come up with a version of the iron cycle, with two examples shown in Fig.
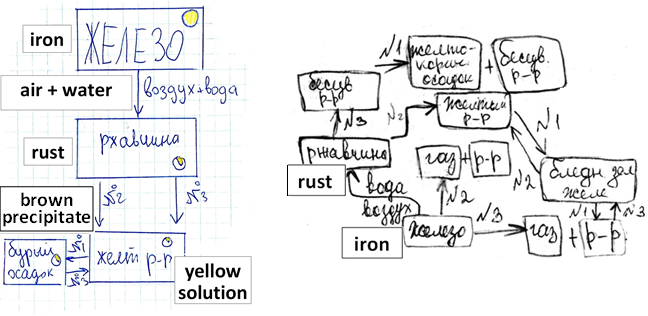
The main feature of these versions is that they don’t specify how to prepare iron because the students don’t know how to do it. This question is asked by the students often, but the answer can be fully understood after the students have mastered the idea of chemical element and locate some of these elements in the familiar cycles, such as the iron, copper, and carbon cycles. In the next year, they can re-read the text about the process of smelting iron, and at this time the question about the second product is expected because the element model can predict it. At this stage a new, more advanced, text can be offered to the students, and they can build the model of the reaction at the next level. So, enter the element model (Fig. 06).
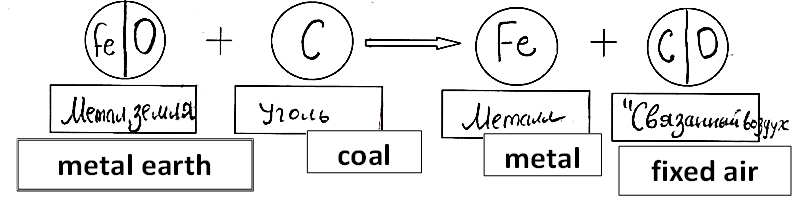
During such work, the iron cycle becomes more complicated (the same happens to all other cycles as well) due to the new questions that have been asked and new experiments that have been planned (Fig. 07).
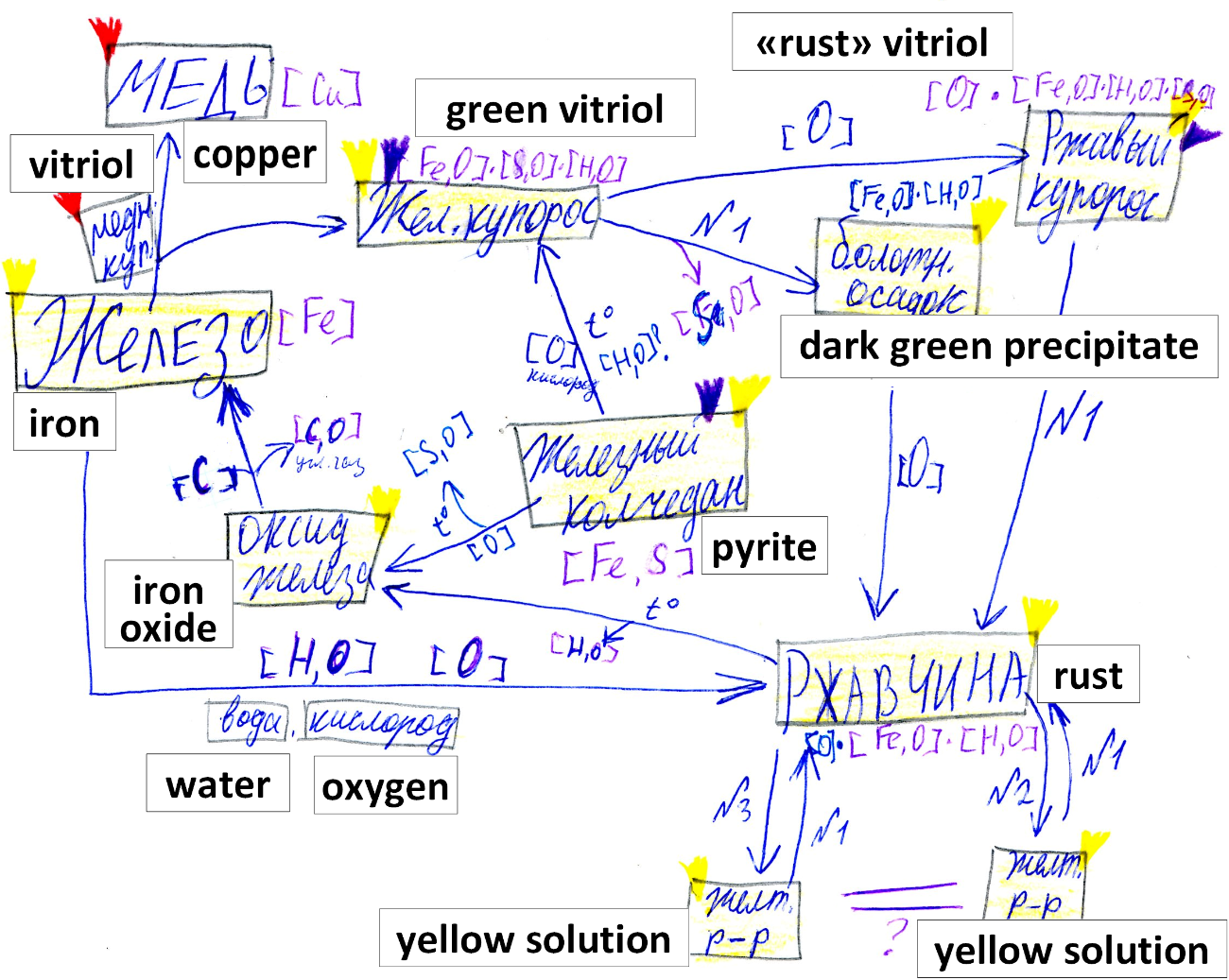
The advanced versions of the cycle give rise to new questions, at which time the new set of texts is offered; the new experiments are planned, and so on. The final stage of this sequence is the atomic model, which is the starting point of the systematic chemistry course for 8-graders.
Learning for Understanding: Benefits of the Approach
Both students and teachers benefit significantly from this approach. For students, the procedural benefits include positive attitude (with chemistry becoming their favorite subject), good motivation and engagement, and absence of problems with homework during the entire course. In addition, our colleagues mentioned that students’ learning initiative grows, especially during our macro-cycle of teaching experiment (Zuckerman, 2010).
On the achievement side, the students’ outcomes include the following:
From a half to three-quarters of the students from our experimental 7th grade enroll every year in the advanced chemistry class for their 8th grade. From the control group, less than one-tenth would want to do the same, choosing a regular class instead.
After our course, the 8-graders from the experimental group solve open-ended indirect formulations of the standard chemistry problems much better than the control group (Fig. 08). It should be mentioned that compared to the control group, the students from the experimental group have two more hours of chemistry per week, spent usually in the lab.
Each year, several of our students (the total of 34 in 4 years) become finalists of various levels of Chemistry Olympiad, from regional up to the national level, and then choose the subject as a major in college.
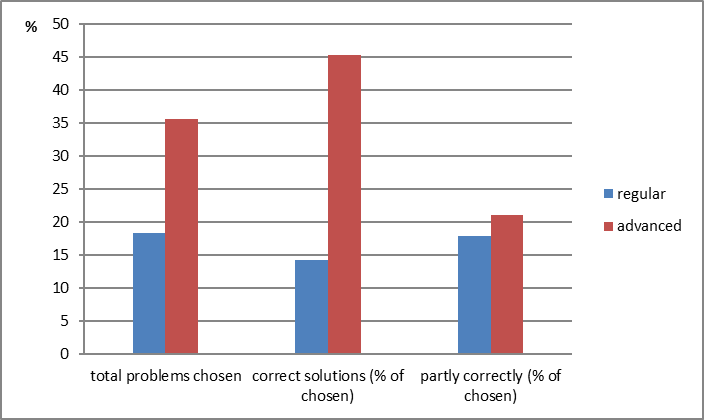
Among the teachers’ benefits, we first note a new understanding of teaching process – it becomes interesting and inspiring. Another observation is a change of opinion about the nature of learning and students’ capabilities. Interestingly, our teachers mentioned many times that in the course of teaching, they have developed a new look on chemistry and its concepts such that the subject has eventually acquired a clear logical structure.
Conclusion
Implementing Developmental Instruction in the science courses for middle school requires many assumptions. Accordingly, the choice of tentative teaching strategies is very wide. Our results convince us that ancient technologies serve as a great cultural-historical context to make the activity of model building meaningful to the students. Carefully chosen historical models work as stepping stones towards modern concepts in natural sciences. Our students show excellent procedural benefits as compared to the control group. Their achievements and the long-term effects of the teaching are promising. We confirm the potential of Developmental Instruction in designing an effective science course and understanding the internals of concept learning.
References
- De Vos, W. & Pilot, A. (2001). Acids and bases in layers: the stratal structure of an ancient topic. Journal of Chemical Education, 78(4), 494-499.
- Matthews, M. (Ed.). (2014). International handbook of research in history, philosophy and science teaching. Springer. doi 10.1007/978-94-007-7654-8_11.
- Meijer, M. R., Bulte, A. M. W. & Pilot, A. (2009). Structure-property relations between macro and micro representations: relevant meso-levels in authentic tasks. In Gilbert, J. K. & Treagust, D. (Eds.), Multiple representations in chemical education. models and modeling in science education. 4, (ch. 9, 195-213). Springer.
- Plomp, T., & Nieveen, N. (Eds.) (2013). Educational design research. Enschede: Netherlands Institute for Curriculum Development (SLO).
- Rubtsov, V. V., & Martin, L. M. W. (Ed.). (1991). Learning in children: organization and development of cooperative actions (M. J. Hall, Trans.). Hauppauge, NY, US: Nova Science Publishers.
- Scheffel, L., Brockmeier, W. & Parchmann, I. (2009). Historical material in macro-micro thinking: Conceptual change in chemistry education and the history of chemistry. In Gilbert, J. K. & Treagust, D. (Eds.). Multiple representations in chemical education. Models and modeling in science education. 4, (ch. 10, p.215-250). Springer.
- Taber, K. S. (2010). Straw men and false dichotomies: overcoming philosophical confusion in chemical education. Journal of Chemical Education, 87(5), 552-558.
- Talanquer, V. (2013). School chemistry: The need for transgression. Science & Education, 22, 1757–1773.
- Van Aalsvoort, J. (2004). Activity theory as a tool to address the problem of chemistry’s lack of relevance in secondary school chemical education. International Journal of Science Education, 26(13), 1635–1651.
- Van Berkel, B., Pilot, A. & Bulte, A.M.W. (2009). Micro-macro thinking in chemical education: why and how to escape. In Gilbert, J.K. & Treagust, D. (Eds.). Multiple representations in chemical education. Models and modeling in science education. 4, (ch. 2, 31-54). Springer.
- Van den Akker J., Gravemeijer, K., McKenney, S., & Nieveen, N. (Eds.) (2006). Educational design research. London: Routledge.
- Vysotskaya, E., Khrebtova, S., & Rekhtman, I. (2015). Linking macro and micro through element cycle: Design research approach for introductory chemistry course for 6-7 grades. LUMAT, 3(3), 409-428.
- Vysotskaya, E., Khrebtova, S., & Rekhtman, I. (2016). A trail over conceptual stepping stones: Ancient technologies and models in introductory chemistry course for 6-7 grades. In ECRICE 2016. Book of Abstracts (p. 184). Barcelona,.
- Vysotskaya, E., Khrebtova, S., Lobanova, A., Rekhtman, I., & Yanishevskaya, M. (2018). Text-lab-model triad for testing ancient technologies: introductory science course for 5- graders. In EAPRIL Conference Proceedings 2017 (pp. 294-309).
- Zuckerman, G. A. (2007). School students age ten to twelve: A “no man's land” in developmental psychology. Journal of Russian & East European Psychology, 45(3), 70-93. doi/abs/10.2753/RPO1061-0405450303.
- Zuckerman, G. A. & Venger, A. L. (2010). Development of learning autonomy by the means of school education. Psychological Science & Education, (4), 77-90.
Copyright information
This work is licensed under a Creative Commons Attribution-NonCommercial-NoDerivatives 4.0 International License.
About this article
Publication Date
23 November 2018
Article Doi
eBook ISBN
978-1-80296-048-8
Publisher
Future Academy
Volume
49
Print ISBN (optional)
-
Edition Number
1st Edition
Pages
1-840
Subjects
Educational psychology, child psychology, developmental psychology, cognitive psychology
Cite this article as:
Vysotskaya, E., Khrebtova, S., Malin, A., Rekhtman, I., & Yanishevakaya, M. (2018). Logic Of Natural Sciences Through Historical Approach: Science For 5-6 Graders. In S. Malykh, & E. Nikulchev (Eds.), Psychology and Education - ICPE 2018, vol 49. European Proceedings of Social and Behavioural Sciences (pp. 741-753). Future Academy. https://doi.org/10.15405/epsbs.2018.11.02.86