Abstract
This paper describes the monitoring works of Integrated Fixed Activated System (IFAS) which are customized to suit its application in river water treatment plant (RWTP) under River of Life (ROL) project that have been monitored by Departments of Irrigation and Drainage (DID) at Klang river and its tributaries. There are seven RWTP selected for monitoring and testing since these RWTP are monitored by the Malaysian Department of Irrigation and Drainage for River of Life Project. Several types of biological carrier in six (6) different RWTPs will be tested for this study. Sampling schedule differ from each RWTP depend on its design and numbers of available chamber and its operation as well as weather condition. Water quality samples from Inlet and Outlet, MLVSS and TVS in the oxidation tank of the RWTP were collected as grab samples for once per month. This IFAS-RWTP can reduce turbidity to acceptable levels, and the effluent water can be achieved to NWQS Class II standard as initial target by the JPS. Further monitoring and analysis are needed to observe the long-term performance in low strength RWTP process in terms of biofilm formation and water quality for better understanding of the IFAS and MBBR processes
Keywords: MBBRIFASbiological water treatment processriverattached growth systemsuspended growth system
Introduction
Since 1978, the Malaysian Department of Environment has been monitoring rivers to establish the criterion and to measure water quality changes in river water quality which includes identifying the type of pollution sources (DOE, 2016). However, many pollutants have emerged rapid changes and these compounds are currently not covered by existing water-quality regulations, have not been studied before, and are thought to be potential threats to environment ecosystems, human health and safety (Ødegaard, 2016). Many problems have occurred which have resulted in the degradation of water quality in every urban river. Nonpoint source pollutants enter the receiving waters during storm events. Typical pollutants found in urban storm runoff include suspended solids, faecal matters, nutrients, chloride, and heavy metals such as aluminium, lead, copper, and zinc (Wang et al., 2011). In addition to increased sediment loads, storm flows flush nutrients likes Nitrogen (N) and Phosphorus (P), sullage, oils, and metals out of the atmosphere and off the pavement (ASCE and Restoration, 2003).
Biological Process for River Quality Improvement Works.
Among the different approaches that have been proposed in these last decades, the technology known as Moving Bed Biofilm Reactor (MBBR) and Integrated Fixed Activated System (IFAS) as shown in Fig
Several studies have produced estimates of biofilm growth and IFAS capabilities of removal toward certain contaminants in municipal and industrial wastewater (Æsøy et al., 1998; Regmi et al., 2011; Hoang et al., 2014), but there is still insufficient data for biofilm’s ability to reduce certain amount of pollutant removal for RWTP including turbidity levels.
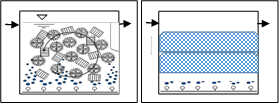
Problem Statement
Despite of data scarcity, the implementation, operation and performance of IFAS systems in removal of pollutants is less reported neither in sewage influent nor river water. Other alternative river treatment such as constructed wetlands and tidal flow wetlands have proven to offer a feasible and sustainable solution to the treatment of polluted river (Clifford et al., 2013; Maria et al., 2013). Although these processes may require significant land areas, they can be energy efficient, minimal maintenance, and effective treatment. Investigation vigorous dynamics of the IFAS system that promotes enhanced biofilm diffusion and performance rates of the RWTP systems at the same time, provide a much higher capacity in the same volume are the main strategies of this study. This raises the question of how to quantify the amount of biofilm formation in RWTP attached growth process and how it will affect the physical performance and capacity of river water treatment system. In the other hand, turbidity removal also been analyzed since high turbid influent river water may disturb the wastewater treatment plant (WWTP) operation and performance (Lu et al., 2011).
Many urban rivers suffer from organic load pollutant. According to Yang (2003), the pollution load of non-point sources in the four major rivers in Korea, non-point sources constitute 22-37% of pollution in terms of BOD level only excluding T-N and T-P. the proportion of nonpoint sources is estimated to be greater if T-N and T-P included. Polluted river can be treated biologically, provided with proper analysis and environmental control. Therefore, Mixed Liquor Suspended Solid (MLVSS) in mg/L/g biomedia will be analyzed
Research Questions
-
What is the pollutant removal efficiency in low loading IFAS in tropical river?
-
What is the biofilm performance rate in the RWTP and how it affects to RWTP performance?
-
How MLVSS in the RWTP influence the performance of each RWTP?
-
How RWTP design factor contribute to RWTP performance?
-
How to predict the kinetic of microbial population in RWTP by using Monod Equation?
Purpose of the Study
This paper describes the application of IFAS and implementation of RWTP as part of ROL project. This will become as a new application in upgrading a polluted river water quality. In future, this research expects to identify the potential of RWTP system in relation to water quality improvement and biofilm technology application for the application in Malaysian river condition. These issues are addressed through a twelve-month monitoring program on seven different location of RWTP. The outcome of this study will be very useful and fundamental data for the design of better biofilm growth, and optimum maintenance of river water treatment projects.
According to Wu (2017), low loading influent strength into biofilm-based biological process system may slower growth of biofilm formation. In doing so, the RWTPs biological performance will be evaluated based on the followings criteria;
Mixed Liquor Volatile Suspended Solid (MLVSS) in the RWTP aerator chamber.
Total Volatile Solids (TVS) on the biological carriers
Scanning Electron Microscope (SEM) for biofilm development
Turbidity Removal Efficiency
Research Methods
There are several main activities to collect data at ROL site for this study.
Site Description
A research site was constructed starting in the year 2013 for ROL Projects around Wilayah Persekutuan Kuala Lumpur and Selangor State. As shown in Fig.
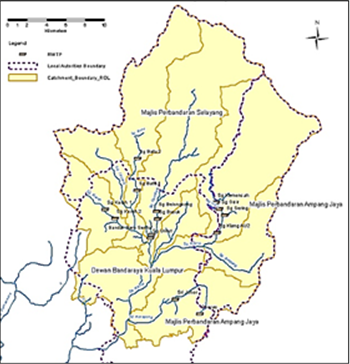
For this study, several types of biomedia were applied in River Water Treatment Plant (RWTP) as a biological process at ROL Project. This study focuses on the evaluation of the biological performance in RWTP. These various removal rates will be evaluated in this study with different biomedia characteristic such as Surface Roughness, Porosity, Pore Size and Specific Surface Area as tabulated in Table
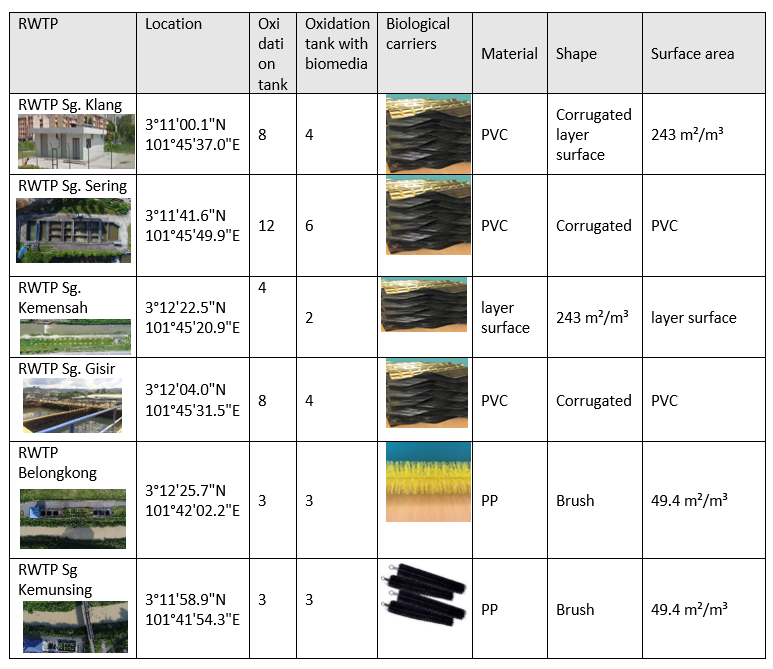
Sampling Procedure
Sampling schedule differ from each RWTP depend on its design and numbers of available chamber and its operation as well as weather condition. MLVSS sampling are referred based on the Figure
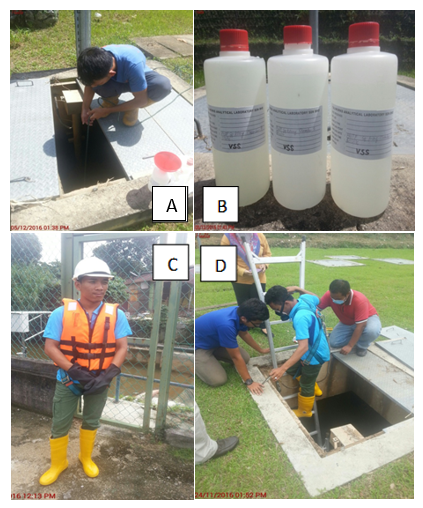
Water quality samples from Inlet and Outlet, MLVSS and TVS in the oxidation tank of the RWTP were collected as grab samples for once per month as shown in Fig 4. They were sampled into a plastic bottle with capacity of 2 litre and sent to certified laboratory for chemical testing as represented in Fig 3. Besides that, an in-situ water quality probe also installed at the bottom of oxidation tank for measuring the physical properties of the temperature, dissolved oxygen and sludge height to monitor the performance of each plant. Once a month influent wastewater, mixed liquor of the different compartments and effluent
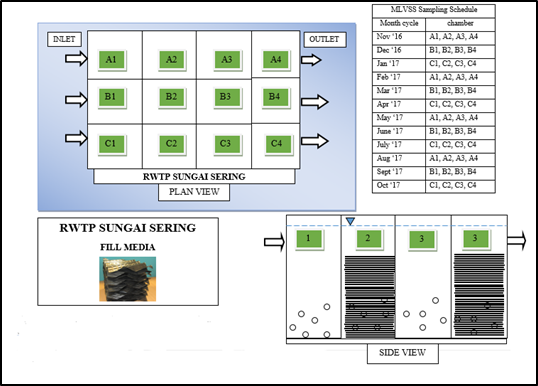
Analytical Method
MLVSS, TVS and Turbidity test have been conducted from grab water sample. The key compounds required to characterize samples were measured according to the Standard Methods (APHA, 2005).
Solid Test for Suspended and Attached growth system
Two parameters recorded to evaluate the biofilm formation in biomedia. The aim of this test is to determine the types of solids either total solids, total fixed solids or total volatile solids in the water samples. According to Bertino (2010) method, biofilm layer formation is measured using total volatile solid (TVS) parameter. To determine TVS, attached biomass fixed in biomass carriers, all seven sample of biomedia carriers were taken out of the reactor and kept in porcelain dishes or beakers with water.
All seven sample of biomedia without biofilm are initially identified and weighted. The biomedia was moved manually using knife and needle until the attached biomass on the carriers were slugged off from the carriers using recorded distilled water. The GFC Whatman’s 1.2 μm filter paper and porcelain dishes were weighted before filtration. Then the solution of biomass and mL water was filtered through a filter paper. The filter paper and porcelain dishes were then kept in the oven at 105 ºC at least for 1 hour followed by desiccation for 20 min and measured weight as “A”. The filter paper was again kept in a furnace at 550 ºC for 20 min followed by desiccation for 20 min and measured weight as “B”. The average biomass was calculated as the average TVS value of the acclimatized carriers. Total volatile solid is mostly organic matter. TVS were calculated as mention in Equation 1.
Where,
A = Weight of filter and porcelain dish + residue after oven 105°C
B = Weight of filter and porcelain dish + residue after ignition
Turbidity
Turbidity level is a standard parameter measured in the river. This paper will analyse the capability of RWTP to remove turbidity in the river water. Plus turbidity is often tested in many previous research for nutrient removal in many biological process (Boye, Falconer, & Akande, 2015; Deng et al., 2016; Zheng et al., 2011).
Findings
TVS is defined as dry weight concentration on the biomedia carriers. Measurement of the TVS on the carriers was carried out to assess whether there was any biofilm growth or not. The measurement of TVS is a roughly approximation of the amount of organic matter present in the solid fraction. The biofilm concentration was calculated between the eights of carriers before and after biomass removal, based on the number of carriers before and their volume in the reactor. TVS were recorded every month starting November 2016. The next stage, for easier prediction of biofilm development, TVS results were calculated in cumulative weight based on experiment duration.
MLVSS test is to determine the operational behaviour and biological inventory of the system. Many researchers applied MLVSS for activated sludge assessment beside COD, TOC, biomass growth, sludge yield and microbial respiration. The measurement of MLVSS is a rough approximation of the amount of organic matter present in the solid fraction. In presence of activated sludge system, it can be an estimation of the biomass concentration (Bertino, 2010). For the month November 2016, there are total 18 MLVSS samples and 14 TVS samples were recorded.
MLVSS Result
A set of data of MLVSS for nine (9) month monitoring as shown in Table
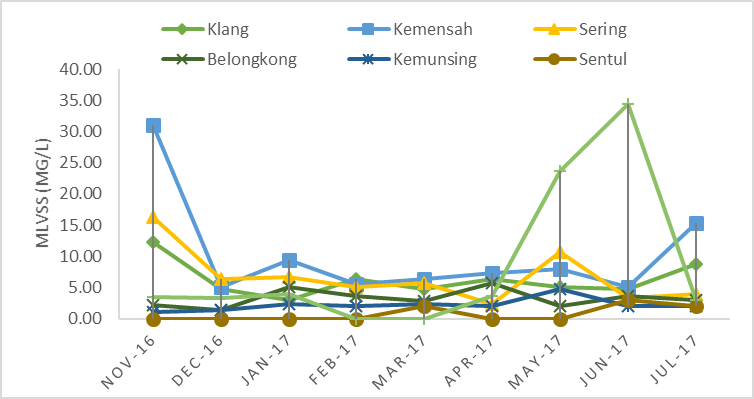
Figure
Although these unsatisfactory MLVSS value outcomes differ from some published IFAS studies, there are several possible explanations for this result. Most previous experimental study did monitor suspended solid value despite of measuring attached biofilm on biological carrier surface to evaluate biomass in the reactor. Pure MBBR had MLVSS concentration in the bioreactor was similar to the influent value (Ahl, Leiknes, & Odegaard, 2006; Di Trapani, Mannina, Torregrossa, & Viviani, 2008; Leyva-Díaz et al., 2014). Suspended biomass concentration was relatively low and constant in total three sampling location for each RWTP, which indicate that biomass growth was homogenous throughout the IFAS biological tanks.
Turbidity Removal
The turbidity removal is shown as in figure
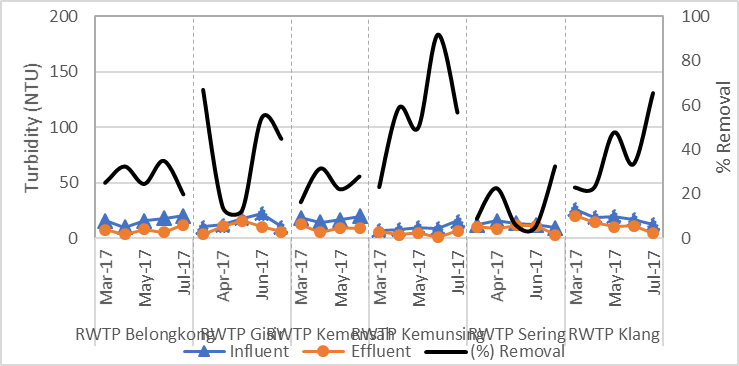
In the present study, the influent and effluent samples are collected from the RWTP location may exhibit large variations in the concentration of organic and inorganic matter leading to unstable results, as detected in the measurement during the initial observation period and the time required to reach steady state condition.
As indicated in the influent level of all RWTPs show a positive relationship between with TSS, meaning that a decrease in TSS concentration correlates with a decrease in turbidity levels. Thus, turbidity could provide a simple estimate of the TSS concentration in the river water despite not directly measuring of TSS in the water (Muhamad, Salim, Lau, Yusop, & Hadibarata, 2016). The efficiency of the RWTPs in reduction of turbidity is impressive. A percentage reduction in turbidity of over 90% could be achieved with an influent turbidity of 8.9 nephelometric turbidity unit (NTU). RWTP Sg Kemunsing shows the highest turbidity removal rate during June 2017 sampling and the lowest removal rate is at RWTP Sg Sering during Jun 2017 which as low as 9.8%. The removal efficiency ranking can be produced by calculating of mean pollutant removal. The average pollutant removal values were RWTP Kemunsing (56.15%) > RWTP Sg Belongkong (54.78%) > RWTP Sg Kemensah (48.92%) > RWTP Sg Gisir (38.68%) > RWTP Sg Klang (38.61%) > RWTP Sg Sering (29.80). This IFAS-RWTP can reduce turbidity to acceptable levels, and the effluent water can be achieved to NWQS Class II standard as initial target by the DID. However, this attached growth biological process suffers from such limitations as the excessive cost of equipment and biomedia and high aeration rate capacity. (Wilson et al., 2012).
Conclusion
Findings show the performances of RWTP are promising under local tropical climate and local pollutant loading. The water quality of the RWTP effluent good and had satisfactorily achieved WQI Class II as targeted. However, the present of attached growth and suspended is consider low and become peculiarity for IFAS system. Dilution by frequent rainfall events in the river catchment during rainy could be the essential factor in affecting the low loading strength of RWTP system of the outflow. The application of IFAS and MBBR in RWTP for ROL project has the potential to improve river pollution as it was proven as a good indicator of a viable strategy of integrated river water management that can be implemented in Malaysia. Further monitoring and analysis are needed to observe the long-term performance in low strength RWTP process in terms of biomass on behalf of both suspended and attached growth and water quality for better understanding of the IFAS processes involved in tropical climate river.
Acknowledgments
The authors acknowledge the UNITEN R&D Sdn Bhd Research Contract (U-EN-CR-17-21) from the Malaysia Department of Irrigation and Drainage (DID) for providing financial support this research project. A part of the instrumentation used in this study was supported by Environmental Engineering Laboratory, Sustainable Technology and Environment Research Group (STEG), Universiti Tenaga Nasional (UNITEN)
References
- Æsøy, A., Ødegaard, H., Hægh, M., Rislå, F., & Bentzen, G. (1998). Upgrading wastewater treatment plants by the use of biofilm carriers, oxygen addition and pre-treatment in the sewer network. In Water Science and Technology, 37, 159–166.
- Ahl, R. M., Leiknes, T., & Odegaard, H. (2006). Tracking particle size distributions in a moving bed biofilm membrane reactor for treatment of municipal wastewater. Water Science and Technology : A Journal of the International Association on Water Pollution Research, 53(7), 33–42.
- APHA. (2005). Standard Methods for the Examination of Water and Wastewater. Washington DC: American Public Health Association.
- ASCE, R. R. S. on U. S., & Restoration. (2003). Urban Stream Restoration. Journal Of Hydraulic Engineering, 129(July), 491–493.
- Bertino, A. (2010). Study on one-stage Partial Nitritation-Anammox process in Moving Bed Biofilm Reactors: a sustainable nitrogen removal. Royal Institute of Technology.
- Boye, B. A., Falconer, R. A., & Akande, K. (2015). Integrated water quality modelling: Application to the Ribble Basin, U.K. Journal of Hydro-Environment Research, 9(2), 187–199. https://doi.org/10.1016/j.jher.2014.07.002
- Clifford, E., Forde, P., McNamara, S., Rodgers, M., & O’Reilly, E. (2013). Performance of Air Suction Flow Biofilm Reactor in Treating Municipal-Strength Wastewater. Journal of Environmental Engineering, 139(6), 864–872. https://doi.org/10.1061/(ASCE)EE.1943-7870.0000682
- Deng, L., Guo, W., Ngo, H. H., Zhang, X., Wang, X. C., Zhang, Q., & Chen, R. (2016). New functional biocarriers for enhancing the performance of a hybrid moving bed biofilm reactor-membrane bioreactor system. Bioresource Technology, 208, 87–93. https://doi.org/10.1016/j.biortech.2016.02.057
- Di Trapani, D., Mannina, G., Torregrossa, M., & Viviani, G. (2008). Hybrid moving bed biofilm reactors: A pilot plant experiment. Water Science and Technology, 57(10), 1539–1545. https://doi.org/10.2166/wst.2008.219
- DID, D. of I. and D. M. (2012). Briefing on River Cleaning Under the River of Life Project.
- DOE, M. of N. R. and E. (2016). Malaysian Environment Quality Report 2016. Putrajaya, Kuala Lumpur.
- Hoang, V., Delatolla, R., Abujamel, T., Mottawea, W., Gadbois, A., Laflamme, E., & Stintzi, A. (2014). Nitrifying moving bed biofilm reactor (MBBR) biofilm and biomass response to long term exposure to 1 °C. Water Research, 49, 215–24. https://doi.org/10.1016/j.watres.2013.11.018
- Leyva-Díaz, J. C., Martín-Pascual, J., Muñío, M. M., González-López, J., Hontoria, E., & Poyatos, J. M. (2014). Comparative kinetics of hybrid and pure moving bed reactor-membrane bioreactors. Ecological Engineering, 70, 227–234. https://doi.org/10.1016/j.ecoleng.2014.05.017
- Lu, S., Niu, X., Ren, Y., & Chen, D. (2011). Application of Downflow-Upflow Biological Aerated Filter in the Pretreatment of Raw Water Containing High Ammonia Nitrogen. Journal of Environmental Engineering, 137(December), 1193–1198. https://doi.org/10.1061/(ASCE)EE.1943-7870
- Mannina, G., Capodici, M., Cosenza, A., Di Trapani, D., & Ekama, G. A. (2017). G.Mannina . , M. Capodici, A. Cosenza, D. Di Trapani, G. A. Ekama. (2017) The effect of the solids and hydraulic retention time on moving bed membrane bioreactors – Part 1: System behaviour. Submitted to Chemical Engineering Journal. Journal of Cleaner Production, https://doi.org/10.1016/j.jclepro.2017.09.200
- Maria, H.-V., Ricardo, S.-C., Javier, M.-V., M. Cruz, V.-B., Josep, M. M., & Eloy, B. (2013). Statistical modelling of organic matter and emerging pollutants removal in constructed wetlands. Retrieved July 2, 2015, from http://ac.els-cdn.com/S0960852411001349/1-s2.0-S0960852411001349-main.pdf?_tid=1d64069e-2096-11e5-83d4-00000aab0f6c&acdnat=1435826661_a17b5ae564e1788ae065945f973fbfc0
- Mohiyaden, H. A. H. A., Sidek, L. M. L. M., Ahmed Salih, G. H., Birima, A. H. A. H., Basri, H., Mohd Sabri, A. F., … Noh, N. (2016). Conventional methods and emerging technologies for urban river water purification plant: A short review. ARPN Journal of Engineering and Applied Sciences, 11(4), 2547–2556.
- Muhamad, M. S., Salim, M. R., Lau, W. J., Yusop, Z., & Hadibarata, T. (2016). The Removal of Bisphenol A in Water Treatment Plant Using Ultrafiltration Membrane System. Water, Air, & Soil Pollution, 227(7), 250. https://doi.org/10.1007/s11270-016-2951-7
- Ødegaard, H. (2016). A road-map for energy-neutral wastewater treatment plants of the future based on compact technologies (including MBBR). Frontiers of Environmental Science and Engineering, 10(4). https://doi.org/10.1007/s11783-016-0835-0
- Regmi, P., Thomas, W., Schafran, G., Bott, C., Rutherford, B., & Waltrip, D. (2011). Nitrogen removal assessment through nitrification rates and media biofilm accumulation in an IFAS process demonstration study. Water Research, 45(20), 6699–708. https://doi.org/10.1016/j.watres.2011.10.009
- Wang, P., Wang, C., Ai, X.-Y., & Yang, C.-Q. (2011). Biofilm characteristics of globular biofilter in the ecological spur-dike and water quality improvement effect on Wangyu River. In 2011 International Conference on Electronics, Communications and Control (pp. 3598–3603). Ningbo, China: IEEE. https://doi.org/10.1109/ICECC.2011.6068074
- Wilson, M., Sandino, J., Cote, T., Brueckner, T., Boltz, J., & Michelsen, D. (2012). Design and Operational Insights of the World ’ s Largest Integrated Fixed Film Activated Sludge ( IFAS ) Process for Low Nitrogen Limits. Proceedings of the Water Environment Federation, (January), 3970–3988. https://doi.org/10.2175/193864712811708248
- Wu, J. (2017). Comparison of control strategies for single-stage partial nitrification-anammox granular sludge reactor for mainstream sewage treatment—a model-based evaluation. Environmental Science and Pollution Research. Springer-Verlag GmbH Germany: Environmental Science and Pollution Research. https://doi.org/10.1007/s11356-017-0230-9
- Yang, H. (2003). Construction of Sound Water Environment and Sustainable Water Supply System in Korea. Seoul, Korea: National Institute of Environmental Research.
- Zheng, H., Zhu, G., Jiang, S., Tshukudu, T., Xiang, X., Zhang, P., & He, Q. (2011). Investigations of coagulation-flocculation process by performance optimization, model prediction and fractal structure of flocs. Desalination, 269(1–3), 148–156
Copyright information
This work is licensed under a Creative Commons Attribution-NonCommercial-NoDerivatives 4.0 International License.
About this article
Publication Date
31 July 2018
Article Doi
eBook ISBN
978-1-80296-043-3
Publisher
Future Academy
Volume
44
Print ISBN (optional)
-
Edition Number
1st Edition
Pages
1-989
Subjects
Business, innovation, sustainability, environment, green business, environmental issues, industry, industrial studies
Cite this article as:
Mohiyaden, H., Sidek, L., Shahrunizam, S., & Hayder, G. (2018). Field Testing And Performance Evaluation Of River Water Treatment Plants. In N. Nadiah Ahmad, N. Raida Abd Rahman, E. Esa, F. Hanim Abdul Rauf, & W. Farhah (Eds.), Interdisciplinary Sustainability Perspectives: Engaging Enviromental, Cultural, Economic and Social Concerns, vol 44. European Proceedings of Social and Behavioural Sciences (pp. 960-970). Future Academy. https://doi.org/10.15405/epsbs.2018.07.02.101