Abstract
The article focused on the consideration of a number of methodological issues related to teaching exploration of deep space to students of the natural science. The paper provides a brief overview of the issues which, in the authors’ opinion, should be taught to students at school and university. In the 21st century, the problem of space exploration is becoming more and more important, so students should be aware of various reasons why at present mankind is not yet ready to solve this ambitious task. The main problem that impedes such travels is cosmic radiation, which is harmful to humans. The article discusses the problems of phosphene generation in astronauts, the effects of cosmic radiation on laboratory animals, the search for materials that can absorb radiation and protect astronauts from harmful external influences. A set of methodological measures that would help to form students' certain ideas about the problems of interstellar flights: analysis of scientific articles, solving problems using real numbers and dependencies, conducting experimental research is discussed. In this paper, we provide examples of tasks that students can solve in order to improve their understanding of high-energy radiation and ways to protect against it. It is concluded that it is necessary to acquaint pupils and students with the problems of flights to near and deep space from the very beginning of studying the natural science disciplines.
Keywords: Teaching materials, deep space exploration, methodology
Introduction
Almost 60 years have passed since the first man went on a space journey. During this time, considerable progress has been made in the development of spacecraft and in ensuring living conditions for astronauts. As a result, long flights into near-Earth orbits have become possible, and flights into space are no longer considered extraordinary. The astronauts simply go on a business trip, during which they do a certain job. Moreover, the problems that used to arise during a long stay of the astronauts in zero gravity, the influence of a long stay in a confined space, and protection from radiation in near-Earth space have already been solved.
One can consider that mankind has mastered near space and it is quite natural that it should desire to move on. Rather, to fly further and explore even more distant corners of outer space and other planets.
The first steps have already been taken in this direction. Since 1960, automatic interplanetary stations have been launched, which have provided information about the characteristics of soil and surface rock, thermal characteristics, atmospheric composition, and the characteristics of the gravitational and magnetic fields of Mars. The next step in the study of this planet is to send robots there, and then people. This will not be just a visit as guests, but real colonisation with the organisation of settlements, the construction of infrastructure and much more. But how soon will this be possible? What needs to be done for this?
Problem Statement
The rest of the paper is not about solving the problem of overcoming the radiation hazard or other harmful cosmic factors. The authors are university teachers, so they are more interested in discussing problems of educating young people, training teachers and expanding their horizons.
It is only natural that students who are interested in space should be watching science fiction films and reading books about the exploration of far regions of the Universe. In this regard, they show a keen interest in this problem. However, our teaching experience shows that students regard such flights merely as most exciting adventures, and are often unaware of the obstacles that currently make such flights impossible.
This impossibility is due to a number of objective reasons, which should be discussed in classes of physics, biology, or other disciplines aimed at the professional training of school teachers.
Research Questions
It is well known that spacecraft that are in near-Earth space are protected from cosmic rays by the magnetic field of the Earth. However, even this protection is not complete. Thus, the astronauts are still exposed to radiation, as is evidenced by their observation of the so-called phosphenes (Narici et al., 2009). This phenomenon was first observed in 1969 by the astronauts who participated in the lunar expedition Apollo 11. NASA studies have concluded that these flares are most likely to be caused by the fast moving charged particles of the cosmic rays that penetrate the Earth's magnetic field, which is weakening with distance from the Earth. Of course, these risks should be taken into account when missions to the Moon and Mars are planned, because beyond the range of the Earth’s magnetic field the crew will be subjected to the powerful impact of high energy cosmic particles.
Scientists believe that the flashes of light perceived by the astronauts are caused by effects of ionizing radiation in the vitreous humour and the retina of the eye (Narici et al., 2009). This group of scientists introduced the first model of the mechanism of the generation of phosphenes. The construction of such a model was extremely important, as it allowed evaluating the risks associated with radiation and its effects on the astronauts in space.
Research into the phenomenon of phosphenes has been conducted by several groups. Currently these flashes are believed to be associated with Cherenkov radiation in the vitreous humour of the human eyes (Tendler et al., 2020). The essence of the Cherenkov phenomenon is that when an ionizing particle moves through a medium at a speed exceeding the phase speed of light in this medium, radiation is produced. This radiation is perceived by the retina. According to some experts (Fuglesang et al., 2004), these flashes of light are not just a phenomenon observed by the eastronauts. In some cases, the phosphenes have caused sleep disorder during the space flight, which has deteriorated the astronauts’ overall health.
The description of the phosphenes refers to cosmic ionizing radiation. Scientists indicate that there are several sources of cosmic radiation. Among them are galactic cosmic rays (GCR) composed of high-energy protons, helium nuclei, and also the nuclei of virtually all elements of the Periodic Table of elements, predominantly carbon and iron; radiation from solar flares; secondary neutron and gamma radiation arising from the interaction of cosmic radiation with the material of the spacecraft and biological tissues, etc. The effects of these emissions are not limited to light flashes. There are also delayed effects such as cataract and cancer (Grigoriev et al., 2013; Krasavin et al., 2012; Ushakov et al., 2018).
Currently, research is underway into the effect of cosmic radiation on biological systems. In particular, this is being done by a group of scientists working on the production in the laboratory of radiation similar to cosmic and its effect on laboratory animals (Grigoriev et al., 2017). These papers present the results of experiments proving that even small doses (20-60 cGy) of accelerated iron ions in animals lead the loss of spatial orientation, while irradiation with accelerated oxygen and titanium ions at doses of 5 cGy leads to cognitive impairment (Marshall-Goebel et al., 2019). Moreover, the effect of these radiation doses is expected to occur within two to three months. Currently, the research is being conducted on laboratory animals, but it may become relevant to astronauts exposed to cosmic radiation over a long period of time.
Understanding the threats to the astronauts during a long space flight, scientists have begun to explore the possibility of creating protection against cosmic radiation.
Moving in this direction, first of all, it is necessary to create detectors of ionizing particles. One such device is the compact detector LIDAL (Light Ion Detector), to be used in the machine ALTEA (Anomalous Long Term Effectson Astronauts) (Narici, et al., 2015, 2017a, 2017b; Rizzo et al., 2018; Walsh et al., 2019). ALTEA was already installed and worked on board the International Space Station (ISS) (2006-2012). It was used to study the radiation situation at the station. The detector design made it possible to detect protons with a temporal resolution of the order of 100 ps. The device is also intended for the preliminary assessment of the possibility of identification of particles in the detector LIDAL-ALTEA on board the ISS. Tests of the device on the Earth have shown that it can detect particles with energies from 90 to 228 MeV with a counting rate of about 109 particles per second (Rizzo et al., 2019).
Research is underway in another direction – the search for materials capable of absorbing radiation and protecting the astronauts from harmful external influences. Attempts have already been made to create special protective suits that can be used in emergency situations on board the ISS (Baiocco et al., 2018). For protection, water-filled clothing is used. To fill it, on-board water is used, which is subsequently processed without waste. Scientists believe that if the experiment succeeds, it will be possible to use its results to develop similar devices for interplanetary flights, including to Mars.
Thus, the current research shows that there is a significant difference between near-Earth and long-distance space expeditions. It is due not only to the preparation of the technical part of the flights, but also to the resolution of biomedical issues. Neither can be solved within the existing concept of preparing long flights. There is a point of view (Szocik & Braddock, 2019) that the preparation of such operations may require extra capabilities of astronauts, which may partly be based on the achievements of genetic engineering, nanotechnology, and robotics. This is a very serious problem that must be solved through a joint effort of scientists, philosophers, and psychologists.
Purpose of the Study
As mentioned above, our main task is to conduct a set of methodological activities with students that would help them develop certain ideas about the problems of interstellar flights. This set involves the use of all the tools available to the teacher: discussing specially selected fragments of scientific papers; solving problems with real numbers and dependences; conducting an experimental study, allowing independent verification of the validity of theoretical data. Of course, it is impossible to use real materials and energies discussed in papers in class, but it is undoubtedly possible to make estimations at the level of models. Examples of this approach are publications dedicated to modelling various flight trajectories to Mars (Singer, 2000). As the authors show, this model can be successfully used in various forms of classes with students (Stinner & Begoray, 2005).
Research Methods
In our opinion, as an experimental task, we can offer the study of the interaction of radiation with matter: the establishment of the material that is most suitable for protection against radiation; the study of a cataract model (as one of the problems arising from exposure to high-energy particles) (Petrova & Sabirova, 2016). The simple experiment described by the authors allows assessing the changes in the image perception that occur in a person with a cataract. The radiation passing through a partially opaque lens becomes not only less intense, but also undergoes a change in its spectral composition (it is shortened by about 20 nm at the blue end of the visible part of the spectrum).
Another experiment that can be carried out within the framework of the students’ laboratory is an experiment to study the absorption of γ-radiation in matter. Recall that the standard version of this study is carried out with a set of metal plates made of different materials (usually aluminium and lead). By changing the thickness of the metal plate placed in the gap between the radiation source and the receiver, the students observe the weakening of the intensity of this radiation. Then, they are invited to construct a graph of the logarithm of the number of the counted pulses n vs. the thickness x of the absorbing layer. Since the number of the counted pulses is proportional to the intensity of the radiation incident on the detector, the absorption coefficient μ can be determined from the slope of the straight line with the use of the law .
We propose supplementing this practical with another task, where water will be used as the absorber of radiation. This does not require the significant modification of the set-up; it has only to be placed vertically, and the vessel can be installed between the radiation source and the detector, and be filled with water to any level. Thus, the students will be introduced to the results of modern experiments that will solve the problem of radiation protection of astronauts (recall the water-filled suits).
The students are also offered problems directly related to the problems discussed above.
Problem 1. According to some researchers (Sannita et al., 2006; Tendler et al., 2020) Cerenkov radiation may be produced in the human eye when a high-energy charged particle crosses the vitreous humour, and the retina and acts as a light sensor, while the brain is able, in turn, to perceive this radiation generated inside the eye. Estimate the minimum momentum (in non-standard units of measurement MeV/c, where c is the speed of light in vacuum), which an electron must have in order for the astronaut to see flashes of light during the space flight even in conditions of very low intensity. The electron moves with a relativistic speed. The refractive index of the vitreous humour is n = 1,33.
Solution. The Cherenkov effect consists in the emission of light when charged particles move in a substance with a speed v exceeding the speed of propagation of light waves (phase velocity) in this medium. Since the phase velocity of light ( is the speed of light in vacuum; is the refractive index of the medium), the condition for the occurrence of the Cerenkov effect is , . Usually, this condition is written in a different form: introducing the parameter we write the condition of the Cherenkov effect as
Since Cherenkov radiation is observed for relativistic particles, we first write the relativistic momentum of the particle:
The minimum momentum corresponds to the minimum value of the parameter β: . Thus, for the minimum momentum we obtain
The calculations are done in non-SI units where the unit of momentum is MeV/c. For the electron, . Dividing both sides of this expression by c, we obtain . Substituting this and = 1.33 into (3) we arrive at
Problem 2. In order to assess the possibility of protecting the crew of a manned mission to Mars from effects of ionizing radiation, calculate the mass of the protective shell of the capsule in which the astronauts will be placed (Douglas & Mellon, 2019). Choose lead ( ) as a protective material and assume that the crew will consist of 6 people. The capsule has the shape of a sphere. There is a volume of about 10 m3 per astronaut, so the total volume is 60 m3. According to scientists, the greatest threat is presented by the solar wind, 90% of which consists of protons with average energies = 5×107 eV. Use the following: charged particles lose energy as a result of Coulomb interaction during collisions with electrons in matter. The rate at which energy is lost in collisions is given by the Bethe-Bloch formula
where E is the particle’s energy, x is the distance travelled by the particle in the medium, n is the number density of electrons in the medium (cm–3), and I is the potential of excitation of the electrons of the medium. The Figure 1 below shows the dependence of the specific energy loss of protons in hydrogen and lead on the initial kinetic energy of the protons (Cazzola et al., 2015).
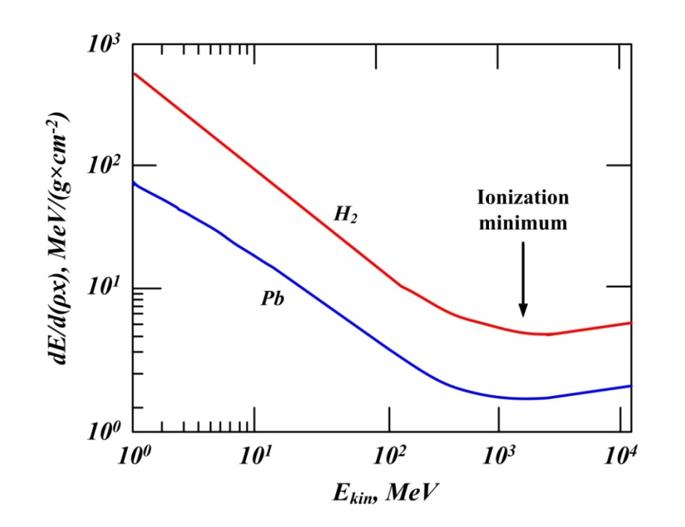
. Given (this is a constant value); ;from the graph, It is necessary to find the mass of the shell of the spacecraft m.
From the formula for the volume of the , we find its radius
Assuming that the thickness of the protective shell is much smaller than the radius of the sphere, we compute the mass , where is the surface area of the sphere, and x is the thickness of the shell.
Since we perform an estimate in this problem, we find the shell thickness from the relation From the conditions of the problem for a particular particle we assume that x takes a specific value. Then Substituting the density of lead, we find . Now we calculate the mass of the shell in SI units:
Thus, the use of the graph greatly simplifies the solution of the problem. There is no need to use the Bethe-Bloch formula, which the students may find rather difficult. Using the data of the graph allows the use of the simplest relations that are familiar even to school students.
Findings
We believe that preparing students for correct understanding of various natural phenomena should begin already at school. The Programme for International Student Assessment is aimed at assessing the awareness of sciences. However, the development of science literacy should not end with graduation. Many graduates go to universities and encounter problems that also require that they should make correct evaluations and informed decisions.
In Ref. 17, the author, the teacher of astronomy N.N. Gomulina showed how the means of the discipline which is being taught allow establishing the validity of the media report about the “huge energy facility that threatens the Earth’s biosphere” and prevent the negative consequences that may arise in society when such reports are read (Gorban & Gomulina, 2015). The reliability of media reports are also discussed in papers by Bulyubash (2010), who created a technique that allows students to examine critically the information flow.
In order to enable students to use what they learn, they need to improve their theoretical knowledge, as well as learn how to use it in various situations. This is what the tasks proposed by the authors are intended to do.
The authors proposed experimental tasks for studying the interaction of ionizing radiation with a substance: establishing the material that is most suitable for protection against radiation; study of the model of cataract (as one of the problems arising from irradiation with high-energy particles), laboratory work on the study of absorption of и radiation and examples of tasks that can be solved with students to enhance their understanding of high-energy radiation and how to protect against them.
Solving problems will allow students to evaluate the energies and velocities that characterize ionizing cosmic radiation, to get acquainted with the off-system units used in carrying out such calculations.
The teaching methods proposed by the authors include carrying out calculations, analyzing the values obtained, working with graphs of functions. In Problem 2, it is shown that the use of the graph greatly simplifies the solution of the problem.
The lessons conducted by the authors based on presented materials showed that this material is interesting to students. Students feel involved in solving serious practical problems. This, of course, increases their motivation to master this material.
Conclusion
Thus, we strongly believe that it is necessary to introduce students at school and university to the problems of deep-space flights already at the initial stage of studying science. This knowledge will help them satisfy their existing cognitive interest, competently evaluate information about space in the media. It is the current schoolchildren that will have to overcome the current obstacles of conquering deep space. The time when the first representatives of the Earth will travel to distant space, when Mars will be colonised, depends first of all on how we are now going to teach physics, astronomy, and biology to our students.
References
Baiocco, G., Giraudo, M., Bocchini, L., Barbieri, S., Locantore, I., Brussolo, E., Giacosa, D., Meucci, L., Steffenino, S., Ballario, A., Barresie, B., Barresi, R., Benassai, M., Ravagnolo, L., Narici, L., Rizzog, A., Carrubba, E., Carubia, F., Neri, G., Crisconio, M., Piccirillo, S., Valentini, G.., Barbero, S., Giacci, M., Lobascio, C., & Ottolenghi, A. (2018). A water-filled garment to protect astronauts during interplanetary missions tested on board the ISS. Life sciences in space research, 18, 1-11.
Bulyubash, B. V. (2010). Science and the media: how to use Internet resources when preparing a report and term paper: guidelines for independent work. Novgorod: NSTU.
Cazzola, E., Calders, S., & Lapenta, G. (2015). Web-based description of the space radiation environment using the Bethe–Bloch model. European Journal of Physics, 37(1), 015605.
Douglas, T. A., & Mellon, M. T. (2019). Sublimation of terrestrial permafrost and the implications for ice-loss processes on Mars. Nature communications, 10(1), 1-9. DOI:
Fuglesang, C., Narici, L., Picozza, P., & Sannita, W. G. (2004). Astronaut light flash survey. ESA-EAC internal report. MSM-am-AHC-GNCRP-001.
Gorban, A. A., & Gomulina, N. N. (2015). A huge energy object and a threat to the Earth’s biosphere. Physics for Schoolchildren, 4, 49-56.
Grigoriev, A. I., Krasavin, E. A., & Ostrovsky, M. A. (2013). On the risk assessment of the biological effects of galactic heavy ions in interplanetary flight. Russian journal of physiology, 99(3), 273-280.
Grigoriev, A. I., Krasavin, E. A., & Ostrovsky, M. A. (2017). To the question of the radiation barrier during manned interplanetary flights. Bulletin of the Russian Academy of Sciences, 87(1), 65-69.
Krasavin, E. A., Ostrovsky, M. A., & Rozanov, A. Yu. (2012). A strobiology: new ideas, problems, prospects. Bulletin of the Russian Academy of Sciences, 82(6), 570-574.
Marshall-Goebel, K., Damani, R., & Bershad, E. M. (2019). Brain physiological response and adaptation during spaceflight. Neurosurgery, 85(5), E815-E821.
Narici, L., Berger, T., Burmeister, S., Di Fino, L., Rizzo, A., Matthiä, D., & Reitz, G. (2017a). Exploiting different active silicon detectors in the International Space Station: ALTEA and DOSTEL galactic cosmic radiation (GCR) measurements. Journal of Space Weather and Space Climate, 7, A18. DOI:
Narici, L., Casolino, M., Di Fino, L., Larosa, M., Picozza, P., Rizzo, A., & Zaconte, V. (2017b). Performances of Kevlar and Polyethylene as radiation shielding on-board the International Space Station in high latitude radiation environment. Scientific reports, 7(1), 1-11. DOI:
Narici, L., Casolino, M., Di Fino, L., Larosa, M., Picozza, P., & Zaconte, V. (2015). Radiation survey in the International Space Station. https://doi.org/
Narici, L., De Martino, A., Brunetti, V., Rinaldi, A., Sannita, W. G., & Paci, M. (2009). Radicals excess in the retina: A model for light flashes in space. Radiation Measurements, 44(2), 203-205.
Petrova, E. B., & Sabirova, F. M. (2016). Study of the Peculiarities of Color Vision in the Course of" Biophysics" in a Pedagogical University. International Journal of Environmental and Science Education, 11(8), 1765-1776. DOI:
Rizzo, A., Berucci, C., De Donato, C., Di Fino, L., Lamastra, F. R., La Tessa, C., Masciantonio, G., Messi, R., Morone C ., Picozza, P., Rovituso, M., Tommasino, F., & Narici, L. (2019, May). LIDAL (Light Ion Detector for ALTEA): a compact Time-Of-Flight detector for radiation risk assessment in space. In Journal of Physics: Conference Series (Vol. 1226, No. 1, p. 012024). IOP Publishing. DOI:
Rizzo, A., Narici, L., Messi, R., Cipollone, P., De Donato, C., Di Fino, L., Iannilli, M., La Tessad, C., Mane, C., Masciantonio, G., Morone, M. C., Nobili, G., Pecchi, D., Picozza, P., Reali, E., Rovituso, M., Tommasino, F., & Vitali, G. (2018). A compact Time-Of-Flight detector for space applications: The LIDAL system. Nuclear Instruments and Methods in Physics Research Section A: Accelerators, Spectrometers, Detectors and Associated Equipment, 898, 98-104.
Sannita, W. G., Narici, L., & Picozza, P. (2006). Positive visual phenomena in space: A scientific case and a safety issue in space travel. Vision research, 46(14), 2159-2165.
Singer, S. F. (2000). To Mars by way of its moons. Scientific American, 282(3) 56-7.
Stinner, A., & Begoray, J. (2005). Journey to Mars: The physics of traveling to the red planet. Physics education, 40(1), 35-45.
Szocik, K., & Braddock, M. (2019). Why Human Enhancement is Necessary for Successful Human Deep-space Missions. The New Bioethics, 25(4), 295-317. DOI: 10.1080/ 20502877.2019.1667559
Tendler, I. I., Hartford, A., Jermyn, M., LaRochelle, E., Cao, X., Borza, V., Hoopes, J., Moodie, K., Marr, B. P., Williams, B. B., Pogua B. W., Gladstone, D. J., & Jarvis, L. A. (2020). Experimentally observed Cherenkov light generation in the eye during radiation therapy. International Journal of Radiation Oncology* Biology* Physics, 106(2), 422-429.
Ushakov, I. B., Shtemberg, A. S., Krasavin, E. A., Bazyan, A. S., Kudrin, V. S., Lebedeva-Georgievskaya, K. B., & Matveeva, M. I. (2018). The effects of cosmic radiation, the combined effects of radiation and other factors of space flight on the functions of the central nervous system in model experiments on animals. Uspehi sovremennoj biologii, 138(4), 323-335.
Walsh, L., Schneider, U., Fogtman, A., Kausch, C., McKenna-Lawlor, S., Narici, L., Ngo-Anh, J., Reitz, G., Sabatier, L., Santin, G., Sihverj, L., Straube, U., Weber, U., & Durante, M. (2019). Research plans in Europe for radiation health hazard assessment in exploratory space missions. Life sciences in space research, 21, 73-82.
Copyright information
This work is licensed under a Creative Commons Attribution-NonCommercial-NoDerivatives 4.0 International License.
About this article
Publication Date
25 September 2021
Article Doi
eBook ISBN
978-1-80296-115-7
Publisher
European Publisher
Volume
116
Print ISBN (optional)
-
Edition Number
1st Edition
Pages
1-2895
Subjects
Economics, social trends, sustainability, modern society, behavioural sciences, education
Cite this article as:
Petrova, E. B., Chulkova, G. M., & Korolev, M. Y. (2021). About Teaching Students Materials Related To Deep Space Exploration. In I. V. Kovalev, A. A. Voroshilova, & A. S. Budagov (Eds.), Economic and Social Trends for Sustainability of Modern Society (ICEST-II 2021), vol 116. European Proceedings of Social and Behavioural Sciences (pp. 1142-1150). European Publisher. https://doi.org/10.15405/epsbs.2021.09.02.127