Abstract
Testing ancient technologies (by the procedures of text analysis and hands-on experiments) is a feasible way to scaffold students’ future promotion in Natural Sciences. This idea became our major hypothesis as we strived to develop a propaedeutic Natural Science curriculum within the Developmental Instruction framework. A student, thus, can acquire the desired concepts through his own activity: these concepts are the guidelines of ancient technologies. One assimilates them through reflection, while he reconstructs these ancient technologies - using special texts devised to serve the purpose. The curriculum builds upon the “spiral” way of developing student’s activity: “text – basic model – experiment – model correction – text”. Our students’ future efficiency in Chemistry compared to that of the control group is analyzed to support our hypothesis. Though both groups are equal in doing Math test, they differ significantly in Chemistry: the experiment groups (we had three cycles, two groups each) perform better.
Keywords: History of science and technologyschool science educationDevelopmental Instruction
Introduction
According to Developmental Instruction (Davydov, 2008), to provide students with solid comprehension of the concepts they study, we have 1) to introduce concepts through students’ own actions and 2) to do so in the context of the concept origin. Within the mentioned framework (which also includes and starts from the Cultural-Historical Approach and Activity Theory) human development is regarded as assimilation of cultural means and meanings. From the early steps of concept formation we have to include children in the meaningful activity adequate to the scientific concepts we aim to provide.
Thus, as researchers on concept formation and also as curriculum designers, we strive to devise special propaedeutic curriculum that will bring students sense and meaning of Natural Sciences. So that when they come to learning separate disciplines (Chemistry, Biology …) they will have questions for the answers they will get.
Problem Statement
The regular way to introduce children to Natural Science domain in primary education is through observations and hands-on experiments, followed by explanations about what had happened (Meyer et al., 2017, Schweingruber et al., 2017). Natural and cultural objects are compared and classified; their common characteristics are extracted to form concepts. According to Davydov (2008), this is the way to form empirical thinking among students. This methodology is at the root of many natural science curricula. Alongside many advices are given on the organization of classroom activities: e.g. on group discussions (Chen et al., 2014).
Another approach is becoming popular nowadays. In its center are the ideas of “STEM-integration” (De Meester et al., 2016; National Research Council, 2014). It is advised to start with practice applications of sciences and to pose contemporary problems on students, so they begin to search for knowledge themselves and are ready for some theory to be poured on them (Prins et al., 2018). For example, Land (2000) suggests using open-ended learning environments to provide students with opportunities to engage in authentic problem solving, such as weather forecasting or water pollution problem. This approach tries to deal with the problem of students’ low motivation towards learning sciences (Krapp & Prenzel, 2011). Gilbert et al. (2010) focuses on devising mental maps – and uses problem contexts (for example making ice-cream in a laboratory) to support mental mapping. We appreciate the idea, but there is a flaw in it: modern technologies are so complicated and so loaded with knowledge that they are too far from the basic scientific concepts. Thus, students may be overwhelmed with special facts and nuances and miss the whole picture.
Both ways – be it observation of nature phenomena, or some real-life problems, researchers note, that it is important to bring together personal and scientific experience (Brickhouse, 1994).
Science education researchers pay great attention to models used (Campbell et al., 2015; Chiu et al., 2015; Wiser & Smith, 2009). Simple illustrative models are introduced to explain nature phenomena (De Vos & Pilot, 2001). Usind analogues is investigated (Cherif et al., 2015). It is considered that children should start with simple and comprehensible nature phenomena before they proceed to scientific concepts.
Concerning texts, that support learning sciences an interesting idea of refutation text is exploited (Mason et al., 2019). They acknowledge and refute reader’s alternative conceptions and introduce scientifi
The last, but not the least: experiments are discussed frequently – from engaging students in real-life modern technologies (as in STEM-approaches) to thought experiments repeating the classic experiments of the past (Gilbert & Reiner, 2000).
We, as researchers and as curriculum designers, continue the traditions of the Soviet Psychology: the Cultural-Historical Approach (Vygotsky, 1962), the Activity Theory (Leontiev, 1978), the Theory of Concept Formation (Galperin, 1992) and the Developmental Instruction (Davydov, 2008). The latter brought the core of the previous theories to the practice of school education. Ideas that we build upon are:
a child’s psychological development depends on the quality of the cultural means he is taught to use (Vygotsky, 1962)
concept formation is through child’s own activity (Leontiev, 1978), external actions are interiorized and form one’s mental actions - and concepts as their guidelines (Galperin, 1992)
reconstruction of the cultural context of concept origin in students’ own activity is the way to form theoretical thinking as opposed to empirical thinking (Davydov, 2008).
Our aim is to form theoretical thinking among students since primary school. That means we want students to be able to look at the processes, objects and concepts they encounter in some science domain from the position of a grown-up specialist of this domain. Thus, when we talk about models, we have to construct those, which will actually mediate students’ actions. Recognizing the importance of experiments, we want them to be consciously planned. We also agree, that students are to use concepts in real-life situations. But we want students to study concepts as they originated from human practice, not only as they can be used. Thus, we exploit the idea of science history as a backbone for science education (Matthews & Matthews, 2014).
Research Questions
What is the special content that will scaffold students’ activity in the desired way?
According to the principles of Developmental Instruction we have devised a special introductory Natural Science curriculum – “NartURE”. We have already researched upon its comparative effectiveness in terms of reading skills immediately after the course (Vysotskaya et al., 2017). Now we want to find out whether it will benefit students’ future promotion in Chemistry – what would be the delayed effect?
Purpose of the Study
Our study has two parts: a) content and curriculum design and b) the experimental part. We will discuss the first part here, as it was our main purpose: to devise special learning environment that will scaffold natural science concepts acquisition.
The main idea that we base upon is: natural science is about human culture rather than about nature itself. The gateway to learning natural science concepts is the reconstruction of special cultural contexts in which these concepts originated and were developed to serve human needs and purposes. The material culture that keeps track of human struggle for survival becomes our new content of natural science curriculum. Thus, students can form concepts as they reflect upon the basic ideas behind ancient technologies, while they reconstruct them – either mentally or actually in the classroom.
Students’ actions that form the spine of curriculum are as follows:
1) A special learning task is introduced: a task to master some meaningful human activity in both aspects: the procedure and the ideas behind it. The task is introduced with and within the cultural context: an urgent human need (warmth, food, safety,...) is chosen.
2) Students work over specially designed texts that provide them problem situation and ways to handle it. Ancient technologies: operations and instruments – are described to give students a clue of how people thought about nature laws, while they made them work to serve their needs.
3) Students construct models to interpret and predict results of actions (either real, or hypothetical) according to the texts.
4) Students test their hypothesis, which they devise with the means of modeling. Hands-on experiments allow to reconstruct (at least partially) the technologies of the past and to check, whether chosen models “work”.
Models appear as a reflection of special positions of discussants and their coordination (see the example with the particle model in the next paragraph). Models mediate text comprehension and at the same time are tools to plan experiments and discuss results.
In fact, students constantly move within a text-model-lab triad (Fig. 1): from text to model, from model to testing their hypothesis in hands-on experiments, than back to correct the model and back to text to read again through a new lens and to proceed to the next cycle. Students work and get results – to manage it, to describe the situation and to discuss results they refer to models and spontaneously enrich model elements with new meanings. Communication is based on the context itself and it is important to make positions of discussants/partners meaningful within the content and context – so that their coordination will have to be mediated by the models.
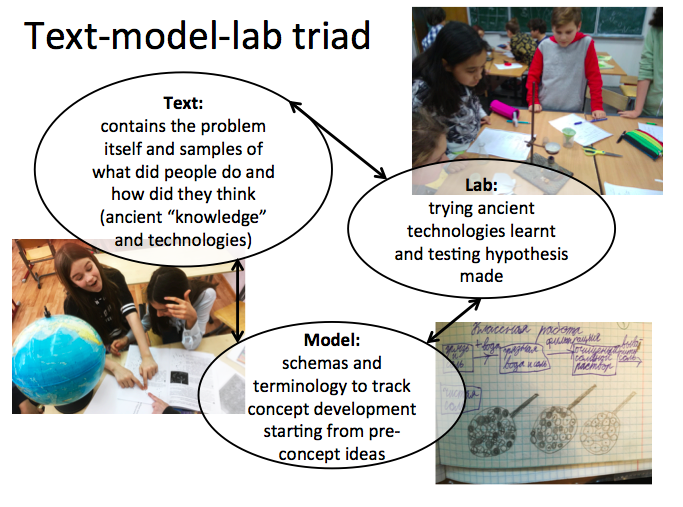
Of course, this kind of curriculum is a challenge to devise, as scientific concepts – not primitive at all – have to be embedded in the content core. It demands 1) a thorough analysis of the history of natural sciences and of human technologies and 2) a psychological analysis of the child’s actions, in which these concepts can be formed, and models to mediate them.
The “NartURE” curriculum contains 8 chapters, each deals with a particular domain of cultural objects that people needed and each scaffolding several future scientific domains. The chapters concern: the occupations of prehistoric people, food, dress, shelter, fire-making, weapons and mettalurgy, medicine, traveller’s instruments.
In the next paragraph, we will give an example from the second chapter: the problem of obtaining pure salt, which exploits the particle model.
Research Methods
Within the educational design research framework (Van den Akker et al., 2006) we have devised “NartURE” curriculum. For seven years this curriculum was taught to 5th graders. Our students’ promotion in natural science domain in grades 7-8 was the main criteria of our curriculum efficiency. We will now present results of the experimental classes compared to control classes, who studied regular natural science curriculum in the 5-6th grades.
Two Moscow schools during 3 years participated in the study, 301 students of the 8th grade in total (15 year-olds): 134 students in the experiment group (having studied “NartURE”) and 167 students in the control group.
We have tested their efficiency in Math to see, that our control and experiment groups are homogenous. In this paper we compare students’ achievements in Chemistry. We have chosen chemistry domain to represent the quality of natural science concepts, as it is one of the most complicated scientific domains, which relies heavily on comprehension.
All diagnostic tests were held according to the regular curriculum.
“NartURE” curriculum
Now we will give an example of the learning environment that our curriculum provides. One of the central topics that exploits the particle model and thus scaffolds future promotion in Chemistry is Salt production. Why did a plain and unpretentious substance drive attention of different people since ancient times? Each nation keeps old tales, riddles and proverbs about salt. People lead wars for salt sources and sometimes salt was valued over gold. How did they get salt?
Students receive copies of ancient texts and illustrations (Fig. 2). Authors of these texts address to future generations and thoroughly go through the whole process of salt-extraction and cleaning. Students begin with the technological flow chart. Starting from the last product – pure salt – they proceed to the raw materials, extracting from the text the sequence of products and the sequence of operations and instruments to transform them. The relation between the desired results (salt has to be dry, pure – without dirt and without other salts, it has to be in granules or some other special form) and the operations performed, becomes students main concern as they move between them in both directions: from attributes to procedure and back.
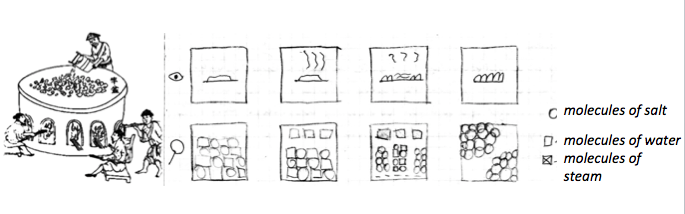
Then students move to the “Lab”-part, as they are going either to test technologies themselves, or to test some hypothesis made – for example, separating salt and mud. They plan to pour water in, than to let it through a filter and evaporate water. The main question thus is: will salt pass the filter? At this point students have to refer to the particle model. The experiment has not yet started.
Salt gives us a vivid example of students’ work with the particle model (Vysotskaya et al., 2015). Students draw particles of substances to model some process of interest, i.e. production of pure salt. The model has two parts: what we can observe in real world and what we would see through a magic lens, that allows to see the smallest particles of substances. As students switch from one part to the other, they obtain the concept of molecule – the smallest particles, that on the one hand preserve the quality of the whole substance and on the other their interposition defines the state of matter: gas, liquid or solid.
The fact that salt appears from water, that seemed absolutely clear, makes us switch to a new language and new hypothesis. To get salt from water we have to think of it as already contained in it. Even though we do not see salt, its attributes are there (the water is salty). Then its invisibility can be modeled as particles, which are far from each other. As we cannot see something that small, the only way to see it – is when these particles gather to form “visible” specks. And that happens, when we evaporate water. The model of particles made this way at last helps to decide (and afterwards prove with an experiment), whether filtration will help to extract salt from salty water. We can also model, what did happen with water molecules, when drops appeared on the cold lid, that we put over the steaming pot of salty water (Fig.3).
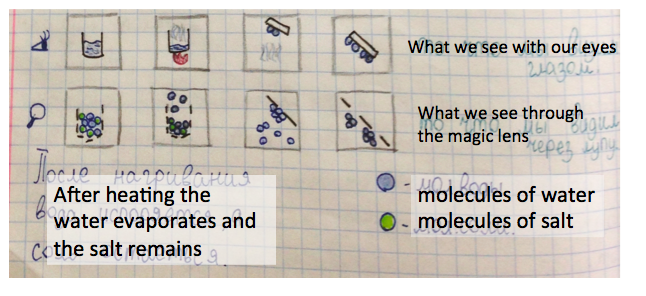
Thus, the conflict between visible and invisible shifts students’ position from an “observer”, who “explains things”, to the one, who can predict things and make them work. Through the particle model students actually understand the ways pure salt was made out of seawater – a task, not a bit simple, though it seems to be. Later on the particle model will be used in many other topics.
Findings
Math tests proved, that groups of students each year were similar (see Table
On the other hand, chemistry tests revealed significant differences (p < 0,01) between groups of each year.
Thus, we can say, that our curriculum, designed according to the Developmental Instruction principles, proved to be effective.
Conclusion
Studying ancient technologies is a feasible way to acquire basic natural science concepts. Models (technological flow chart, particle model and others) help students to reconsider their personal everyday experience and naïve concepts that they already head. Comparing modern technologies with their archaic ancestors students understand modern procedures deeper as they grasp basic principles that stay the same through generations. They also begin to respect past generations and acknowledge the gigantic work done to bring humanity to the current state of technology and science. It is a common myth among students nowadays, that people before were not as clever, as modern are – and it is great to see students overcoming it. We strive to fill each and every concept of natural science curricula in secondary and high education with sense and meaning for students. We hope, that after the introductory curriculum that we devised, based on the principles of Developmental Instruction, students will always appeal to human cultural activity and actions behind the basic concepts and ideas of Sciences.
The last but not the least, we would like to underline the cultural identification, which this curriculum scaffolds. It is not the science, which is “dragged” to students and reshaped to suit their personal needs. Instead, students are introduced to the context of concept origin. They accept human urgent needs of the past as their own – and thus identify themselves with the most bright and worthy people (not only separate individuals, who’s names we know) in the history of human culture and science, who solved these problems and moved humanity ahead.
References
- Brickhouse, N. W. (1994). Children's observations, ideas, and the development of classroom theories about light. Journal of Research in Science Teaching, 31(6), 639-656.
- Campbell, T., Oh, P. S., Maughn, M., Kiriazis, N., & Zuwallack, R. (2015). A review of modeling pedagogies: Pedagogical functions, discursive acts, and technology in modeling instruction. Eurasia Journal of Mathematics, Science & Technology Education, 11(1).
- Chen, Y-C., Lin, J-L., & Chen, Y-T. (2014). Teaching Scientific Core Ideas through Immersing Students in Argument: Using Density as an Example. Science Activities: Classroom Projects and Curriculum Ideas, 51(3), 78-88
- Cherif, A. H., Siuda, J. E., Roze, M., Movahedzadeh, F., & Gialamas, S. (2015). Enhancing Student Understanding Through Analogies in Teaching Science Concepts: Teacher and Faculty Perspectives. Pinnacle educational research and development, 3(3). https://www.pjpub.org/perd/perd_185.pdf
- Chiu, J. L., DeJaegher, C. J., & Chao, J. (2015). The effects of augmented virtual science laboratories on middle school students' understanding of gas properties. Computers & Education, 85, 59-73.
- Davydov, V.V. (2008). Problems of Developmental Instruction. Nova Science Publishers (Original work published in 1986).
- De Meester, J., Knipprath, H., Thielemans, J., De Cock, M., Langie, G., & Dehaene, W. (2016). Integrated STEM in secondary education: A case study. In Nuovo Cimento C: colloquia and communications in physics (Vol. 38, No. 03). Societa Italiana di Fisica (SIF).
- De Vos, W., & Pilot, A. (2001). Acids and bases in layers: The stratal structure of an ancient topic. Journal of Chemical Education, 78(4), 494.
- Galperin, P. I. (1992). Stage-by-stage formation as a method of psychological investigation. Journal of Russian and East European Psychology, 30(4), 60–80.
- Gilbert, J. K., & Reiner, M. (2000). Thought experiments in science education: potential and current realization. International Journal of Science Education, 22(3), 265-283.
- Gilbert, J. K., Bulte, A. M. W., & Pilot, A. (2010). Concept Development and Transfer in Context‐Based Science Education. International Journal of Science Education, 33(6), 817–837
- Krapp, A., & Prenzel, M. (2011). Research on interest in science: Theories, methods, and findings. International journal of science education, 33(1), 27-50.
- Land, S. M. (2000). Cognitive requirements for learning with open-ended learning environments. Educational Technology Research and Development, 48(3), 61-78.
- Leontiev, A.N. (1978). Activity, consciousness, and personality. Prentice Hall. (Original work published 1975).
- Mason, L., Zaccoletti, S., Carretti, B., Scrimin, S., & Diakidoy, I. A. N. (2019). The role of inhibition in conceptual learning from refutation and standard expository texts. International Journal of Science and Mathematics Education, 17(3), 483-501.
- Matthews, M. R., & Matthews, M. R. (Eds.). (2014). International handbook of research in history, philosophy and science teaching. Springer.
- Meyer, J. W., Kamens, D., & Benavot, A. (2017). School knowledge for the masses: World models and national primary curricular categories in the twentieth century (Vol. 36). Routledge.
- Council, T. A., & National Research Council. (2014). STEM learning is everywhere: Summary of a convocation on building learning systems. National Academies Press.
- Prins, G. T., Bulte, A. M., & Pilot, A. (2018). Designing context-based teaching materials by transforming authentic scientific modelling practices in chemistry. International Journal of Science Education, 40(10), 1108-1135.
- Schweingruber, H., Beatty, A., & National Academies of Sciences, Engineering, and Medicine (2017). Seeing students learn science: Integrating assessment and instruction in the classroom. National Academies Press.
- Van den Akker, J., Gravemeijer, K., & McKenney, S. (2006). Introducing educational design research. In Educational design research (pp. 15-19). Routledge.
- Vygotsky, L. S. (1962). Thought and Language. 1934. (translated by. E. Hanfmann & G. Vakar). MIT P.
- Vysotskaya, E., Khrebtova, S., & Rekhtman, I. (2015). Linking macro and micro through element cycle: Desing research approach for introdutory chemistry course for 6-7 grades. LUMAT (2013–2015 Issues), 3(3), 409-428.
- Vysotskaya, E., Rekhtman, I., Lobanova, A., & Yanishevskaya, M. (2017). Text-lab-model triad for testing ancient technologies: introductory science course for 5-graders. In EAPRIL 2017 Conference Proceedings, 294.
- Wiser, M., & Smith, C. L. (2009). Learning and Teaching about Matter in Grades K–8: When Should the Atomic-Molecular Theory be Introduced? In International handbook of research on conceptual change (pp. 233-267). Routledge.
Copyright information
This work is licensed under a Creative Commons Attribution-NonCommercial-NoDerivatives 4.0 International License.
About this article
Publication Date
15 November 2020
Article Doi
eBook ISBN
978-1-80296-093-8
Publisher
European Publisher
Volume
94
Print ISBN (optional)
-
Edition Number
1st Edition
Pages
1-890
Subjects
Psychology, personality, virtual, personality psychology, identity, virtual identity, digital space
Cite this article as:
Vysotskaya, E., Yanishevskaya, M., Lobanova, A., Khrebtova, S., & Rekhtman, I. (2020). Ancient Technologies As A Gateway To Study Natural Sciences. In T. Martsinkovskaya, & V. Orestova (Eds.), Psychology of Personality: Real and Virtual Context, vol 94. European Proceedings of Social and Behavioural Sciences (pp. 846-854). European Publisher. https://doi.org/10.15405/epsbs.2020.11.02.102