Abstract
This paper concerns the rational application of blended learning (BL) for the three cycles of engineering education. The methodology for the design of BL-engineering programmes with optimal combination of the on-campus and online training is presented. The methodology is based on the CDIO: Conceive-Design-Implement-Operate approach initially developed for undergraduate engineering education and further evolved for graduate (FCDI: Forecast-Conceive-Design-Implement) and postgraduate (FFCD: Foresight-Forecast-Conceive-Design) engineering education (CDIO-FCDI-FFCD models). The optimization criterion is the maximum efficiency of achieving intended learning outcomes (LOs), required for complex (CDIO), innovative (FCDI) and research (FFCD) engineering activity. The design of BEng, MSc and PhD BL-engineering programmes involves identifying how far the digital transformation in engineering education can be realized when training the graduates for the three types of engineering activities (complex, innovative, research) taking into consideration graduate’s competencies required to work at various stages (Foresight-Forecast-Conceive-Design-Implement-Operate). The design methodology provides an opportunity to determine the rational level of digitalization and the application of on-campus and online training for various BL-engineering programmes. Specific examples show that a rational component of online training in the implementation of BL-engineering programmes can vary from 40% (undergraduate) to 70% (postgraduate). The rational increase in the online component increases the academic mobility of students, while ensuring the quality of their preparation for professional activities.
Keywords: Engineering educationblended learningoptimizationCDIO approach
Introduction
The digital revolution that has been occurring since the middle of the last century is rapidly changing strategies and technologies in the field of education, including higher education (HE) and engineering education (EE) in particular (Aleksakov, 2017; Alexandrov, Fedorov, & Medvedev, 2013; Chuchalin, 2018b; Ivanov, Kaybiyaynen, & Miftakhutdinova, 2017; Kondakov, 2017; Veshneva & Singatulin, 2016). Thanks to the use of online technologies, distance learning has become an active substitute for traditional teaching at the university. A lot of discussions have been devoted to the issues of application of online training and distance learning in HE, as well as to comparative analysis of their advantages and disadvantages in relation to the classical on-campus education (Erstad, 2017; Khalid, Shquier, & Alsmadi 2016; Miller 2014; Reis, Amorim, Melão, & Matos, 2018).
The main advantage of classical teaching on the university campus is the possibility of a harmonious combination of the education and training of the student to prepare him (her) for future professional activity (development of professions competences), and his (her) socialization (acquiring the experience of communication and social life) by direct (face-to-face) contact with teacher and other students in the classroom and out of it, as well as student participation in sports, cultural and other extracurricular activities that contribute to the student personal development. With the use of distance learning, this combination is almost impossible. At the same time, distance learning is more flexible. A student in most cases can choose for himself (herself) the convenient pace and rhythm of learning, as well as the options in mastering the online programme. However, he (she) needs a special motivation and responsibility. The student develops self-discipline, which is more rigorous and sustainable than it is achieved when studying on the university campus, where the majority of classes are regulated by the timetable (Erstad, 2017; Miller, 2014).
Online training using the Internet has great potential in terms of attracting global information resources and can be significantly richer in content than the on-campus training. Creating e-resources for distance learning (video and audio material, interactive e-textbooks, VR-training programs and simulators, etc.) requires significant expenditure. However, the implementation of online training, as a rule, is cheaper than the traditional one (https://potomac.edu/learning/online-learning-vs-traditional-learning/). Many experts consider that the results of online training are not inferior to the results of studying at the university campus. Other experts are more restrained in assessing the capacity of online technologies. They believe that not all disciplines can be implemented in the form of online courses. Nevertheless, e-learning enthusiasts insist that there are no restrictions for on-line training and distance learning in the era of the digital revolution (Gleason, 2018; Morshed, 2017; Ossiannilsson, Williams, Camilleri, & Brown, 2015). The current stage in the development of online education is associated with the creation of mass open online courses (MOOCs) in various modifications. The emergence of MOOCs served as a new impetus to the development and application of online training and distance learning in HE including EE. The expansion of the application of online training increases the academic mobility of students, which is a significant advantage over on-campus training.
Problem Statement
Discussions related to the use of online training and distance learning in HE are particularly relevant for EE. This is due to the special requirements for the material support of engineer training (the use of real equipment, devices, instruments and materials) and the need for student practical work in laboratories and workshops to acquire hands-on experience. These requirements are limiting factors for online EE. However, online training is actively used in EE in the US and European universities at all levels. Some of engineering programmes are implemented completely online, while others are partially (visits to laboratories, internship, public defense of dissertations, etc. are required). It is important to note that online engineering programmes in the US are accredited by ABET, the world leader in quality evaluation of EE, with the use of the same criteria as for traditional engineering programme accreditation (http://www.abet.org/accreditation/new-to-accreditation/online-programs/#online).
Coming back to the problem of using laboratories and workshops in engineer training, as well as importance of students' acquisition of practical skills in working with real equipment, devices and instruments, it should be noted that in online training this problem can be partially solved by using VR-technologies and remote access to laboratory equipment (Richert, Shehadeh, Willicks, & Jeschke, 2016). Nevertheless, most researchers and practitioners of EE believe that the best option for engineer training is the application of the blended learning method, using the advantages of both online and on-campus modes, including hands-on experience (Graham, 2018; Jones & Chew, 2015; Kamp 2016).
When designing and implementing engineering programmes using the BL method, it is possible to optimally combine online and on-campus training to achieve a synergistic effect and a real improvement in the quality of graduate preparation for professional activities. Depending on the curriculum and the content of the courses, different variants of combination of online and on-campus training may be used (Alammary, Sheard & Carbone, 2014; Sousa & Álvaro, 2018; Brown 2015; Douglas, Ionescu, Petrolito, & Mainali, 2016; Galvis, 2018; van Puffelen, 2017). The main idea is to make maximum use of the advantages of each of them (Brown, 2015; Douglas et al., 2016; Galvis, 2018; Graham, 2018). The potential for the application of the BL method is related to its optimization taking into account the prospects of achieving intended LOs of engineering programme graduates at different levels of HE. The problem is to find appropriate models for the three-cycle EE LOs definition and achievement.
Research Questions
For the design of three-cycle engineering programmes with maximum use of the advantages of on-campus and online training when achieving varied LOs, it is promising to apply the CDIO approach, adapted to the specifics of Bachelor’s, Master’s and Doctor’s training (CDIO-FCDI-FFCD models). The CDIO model focuses on the LOs required for complex engineering activity of graduates at four stages of the life cycle of products, processes and systems: “Conceive”, “Design”, “Implement” and “Operate” (Crawley, Malmqvist, Ostlund, Brodeur, & Edström, 2014). The CDIO approach has proved its effectiveness for the design of BEng-programmes, and many universities around the world successfully implement the CDIO model in practice (http://cdio.org/). The CDIO approach has become also of great importance for the development of the theory of EE (Edstrom, 2017). The FCDI model and FFCD model were developed recently as a result of the evolution of the CDIO approach (Chuchalin, 2018a). Based on the core competences required for innovative engineering activity the FCDI (Forecast, Conceive, Design, Implement) model was developed for the design of engineering MSc-programmes. Based on the core competences required for research engineering activity the FFCD (Foresight, Forecast, Conceive, Design) model was developed for the design of engineering PhD-programmes. The CDIO-FCDI-FFCD triad corresponds to the features of the division of labor in the engineering profession and responds to the challenges of the ongoing fourth industrial revolution (Chuchalin, 2018c; Gleason, 2018; Kamp, 2016; Schwab, 2016).
Purpose of the Study
To design BEng, MSc and PhD-engineering programmes planned for delivery by the BL method, it is necessary to start with assessing the possibility and feasibility of using on-campus and online training of students to prepare them for complex, innovative and research engineering activities, respectively. In the conditions of trendy “universal digitalization”, the programme developers will certainly strive to increase the online training component. However, it is very important to carefully study and assess the rationality of using a particular technology in terms of achievement of the graduate LOs required for working at each stage of complex, innovative or research engineering activity. Below is a methodology for applying CDIO-FCDI-FFCD models to the design of three-cycle engineering programmes for delivering by the BL method and maximizing the benefits of on-campus and online training. It is assumed that online training via the Internet is carried out exclusively at a time when students are out of campus. The use of e-resources to support the learning process when teaching on the university campus is not considered online training. The idea of the methodology is to optimize online and on-campus training components in order to achieve intended LOs with maximum efficiency and to expand the opportunities for distance learning while mastering the BL-engineering programmes of the three cycles.
Research Methods
To design an engineering programme, university developers usually invite experts - representatives of stakeholders, and first of all, researchers and practitioners from industry. To design three-cycle BL-engineering programmes the university developers and experts should start with identifying how far the digital transformation in EE can be realized when training the graduates for the three types of engineering activities (complex, innovative, research). The decision should be based on the assessment of the possibilities of reaching graduates’ competencies (programme LOs) required to work at various stages of engineering activity, by online training. Then the modular programme structure (courses on natural sciences and mathematics, humanities, engineering sciences, research, internship, etc.) should be designed. When the structure of the programme is designed by modules, and their general content is defined, the developers and experts should determine which of the programme modules and in what part should be delivered on-campus, and which of the modules and in what part can be delivered using the Internet and online tools. To do this it is important to decompose the lists of the programme LOs for detailed LOs (knowledge, skills, attitudes) of the graduates of BEng, MSc and PhD programmes and to identify the content of the curriculum elements that ensure the achievement of the intended LOs. When on-campus and online training components of the programme modules are determined, developers and experts should identify which types of learning activities (lectures, seminars, labs, workshops, etc.) and in what part should be used on-campus, and which types of the online tools, and teaching & learning (T&L) material (videos, virtual labs, e-texts, simulation, webinars, etc.) should preferably be used for distance learning. For that it is important to identify which types of on-campus training and which online tools are the most effective for achieving particular LOs of the graduates of BEng, MSc and PhD-programmes. Finally, the level of the digital transformation in each of the three cycles of EE can be defined. The proposed methodology is presented below on the basis of the pilot three-cycle BL-engineering programmes “Technologies of Food Production from Plant Raw Materials” (specialization: Oil & Fat, and Perfume-Cosmetic Products)” designed in Kuban State Technological University (KubSTU).
Findings
Digital Transformation in the 1st cycle of Engineering Education
A CDIO undergraduate programme as the 1st cycle of EE is based on the principle that product, process, and system lifecycle development and deployment are the appropriate context for basic engineering education. Conceiving – Designing – Implementing - Operating is the model of the entire product, process, and system lifecycle (Crawley, Malmqvist, Ostlund, Brodeur, & Edström, 2014). For the design of a BEng-programme it is important to understand the specifics of the graduates’ training for complex engineering activity. Considering the content of the work at each stage of complex engineering activity (C-D-I-O), as well as the required graduates’ competencies, the CDIO-programme developers and experts estimate the possible ratios between on-campus and online training components, taking into account the opportunity of achieving intended LOs. The diagram (Figure
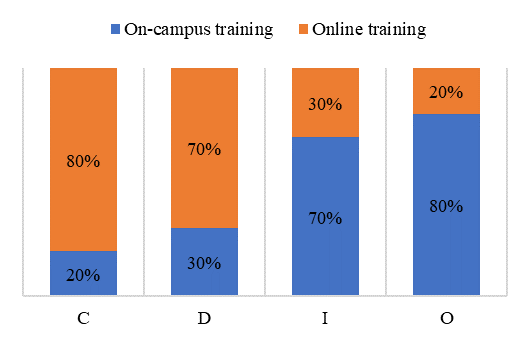
As follows from the diagram in the Figure
Further, it is possible to design each curriculum element (course) within each BEng-programme module. The optimal ratios between online and on-campus T&L components for the delivery of each course can be determined taking into consideration the detailed course LOs and types of the on-campus and online learning activities providing achievement of the intended LOs. It was founded that as a result of digital transformation, the pilot CDIO-programme can be mastered by BEng-students using online distance learning by 40%. This will allow students to have more freedom in terms of individualization of learning, providing academic mobility and the use of other benefits of distance learning.
Digital Transformation in the 2nd cycle of Engineering Education
A FCDI graduate programme as the 2nd cycle of EE is based on the principle that innovative product, process, and system design and development lifecycle: Forecasting – Conceiving - Designing - Implementing is an adequate competence model for MSc degree in engineering (Chuchalin, 2018a). Considering the content of the work at each stage of innovative engineering activity (F-C-D-I), as well as the required graduates’ competencies, the FCDI-programme developers and experts estimate the possible ratios between on-campus and online training components, taking into account the opportunity of achieving the intended LOs. The diagram (Figure
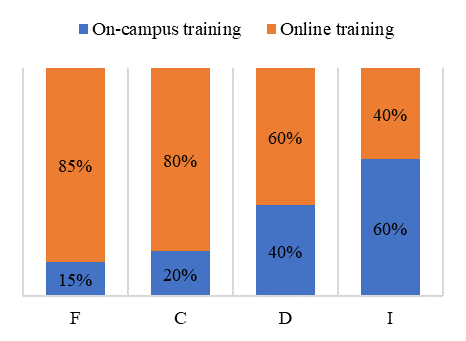
As follows from the diagram in the Figure
Based on the estimations and the results of critical assessment of the opportunities of achieving intended LOs of each module, the developers and experts rationally design each curriculum element and determine optimal ratios between online and on-campus T&L components. It was founded that as a result of digital transformation, the pilot FCDI-programme can be studied remotely by MSc-students by 60%. This gives students a lot of benefits, including possibility to combine university study with practical work in industry.
Digital Transformation in the 3rd cycle of Engineering Education
A FFCD postgraduate programme is based on the principle that creation of scientific basis for the development and design of innovative product, process, and system lifecycle: Foreseeing – Forecasting - Conceiving - Designing is an adequate competence model for PhD degree in engineering (Chuchalin, 2018a). Considering the content of the work at each stage of the research engineering activity (F-F-C-D), as well as the required graduate’s competencies, the developers and experts estimate the preferable ratios between on-campus and online training components of the FFCD-programme, taking into account the opportunity of achieving the intended LOs. The diagram (Figure
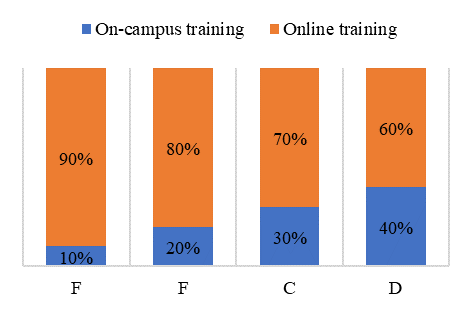
As follows from the diagram in the Figure
Based on the estimations, it is possible to design each module and curriculum element within PhD-programme and to determine optimal ratios between the online and on-campus T&L components. It was founded that as a result of digital transformation, the intended LOs of the pilot FFCD-programme can be achieved by PhD-students by 70% out off campus. This will enable students to move around in the course of study and to do research not only in their university, but also in other leading research centers, as well as to participate in domestic and international conferences to share knowledge. All this will significantly improve the quality of the PhD-student research work and prepare graduates for future research activities in the era of the industrial revolution and the digital economy.
Conclusion
Blended learning, which is a combination of on-campus and online training, is increasingly used in three-cycle engineering education. The potential for applying the BL method is related to its optimization based on the criterion that is the maximum efficiency of achieving intended LOs at the required level using on-campus and online training. To increase the component of online training in the BL method and to maximize the benefits of distance learning, it is necessary to increase the level of digitalization of engineering programmes. The methodology presented in this paper enables to assess the rational level of digital transformation in EE in each of the three cycles of graduate training for complex, innovative and research engineering activity, respectively. The methodology is based on the CDIO-FCDI-FFCD models developed taking into account the peculiarities of the division of labor in the engineering profession and appropriate context of complex, innovative and research engineering activity. The methodology assumes an analysis of graduates’ competencies required at different stages (Foresight, Forecast, Conceive, Design, Implement, Operate) of engineering activity and assessment of the opportunities to achieve intended LOs by the students of BEng, MSc and PhD-programmes with the use of on-campus and online training. As a result of the decomposition of programme LOs the modular structure and content of the three-cycle engineering programmes are developed. The optimal ratios between online and on-campus training components are defined for each module and curriculum element based on the criterion to achieve intended LOs with maximum efficiency. Finally, the rational level of digital transformation in the three cycles of EE for application of the BL method are defined. The methodology given in this paper has been tested in Kuban State Technological University. Based on the examples of the pilot three-cycle engineering programmes in the field of food production technologies, it was shown that due to rational application of the BL method a CDIO-programme can be mastered by BEng-students using distance learning by 40%, a FCDI-programme can be studied remotely by MSc-students by 60%, and a FFCD-programme intended LOs can be achieved by PhD-students by 70% out off campus. The increase of the online training components enables students to have more freedom in terms of individualization of study and the use of other benefits of distance learning. The methodology presented in this paper is recommended for use by EE practitioners dealing with the development of three-cycle engineering programmes designed for delivery by BL method. The methodology is also subject to further enhancement through more detailed research in the field of the BL method rational application with the help of the CDIO-FCDI-FFCD models.
Acknowledgments
The authors are grateful to KubSTU academic staff for the collaboration when developing the pilot CDIO-FCDI-FFCD programmes.
References
- Alammary, A., Sheard, J., & Carbone, A. (2014). Blended Learning in Higher Education: Three Different Design Approaches. Australasian Journal of Educational Technology, 30(4), 440-454.
- Aleksakov, A. M. (2017). Chetvertaya promishlennaya revolutsiya i modernizatsiya obrazovaniya: megdunarodniy opit [The Fourth Industrial Revolution and the Modernization of Education: International Experience]. Strategic Priorities, 1(13), 53 -70. [in Rus.].
- Alexandrov, A. A.., Fedorov, I. B., & Medvedev V. E. (2013). Ingenernoye obrazovaniye segodnya: problem i resheniya [Engineering Education Today: Problems and Solutions]. Higher Education in Russia. 12, 3-8. [in Rus.].
- Brown, M. G. (2015, June), Mapping the Study of Blended Learning in Engineering Education Paper presented at 2015 ASEE Annual Conference & Exposition, Seattle, Washington.
- Chuchalin, A. (2018a). Evolution of the CDIO approach: BEng, MSc and PhD level. European Journal of Engineering Education.
- Chuchalin A. I. (2018b). Ingenernoye obrazovanie v epokhu industrialnoy revolutsii i tsifrovoy economiky [Engineering education in the epoch of industrial revolution and digital economy]. Higher education in Russia, 10, 47-62. [in Rus.].
- Chuchalin, A. I. (2018c). Modernizatsiya trekhurovnevogo visshego obrazovaniya na osnove FGOS 3++ i CDIO++ [Modernization of the three-cycle Engineering Education based on FSES 3++ and CDIO++]. Higher Education in Russia, 28(4), 22-32. [in Rus.].
- Crawley, E., Malmqvist, J., Ostlund, S., Brodeur, D., & Edström, K. (2014). Rethinking Engineering Education, the CDIO Approach, Second Edition. New York, NY: Springer.
- Douglas, K. T., Ionescu, D., Petrolito, J., & Mainali, B. (2016). Is Blended Learning the Answer to Enhance Learning of Engineering Students? In A. Rahman, & I. Vojislav (Eds), Proceedings of International Conference on Engineering Education and Research (pp.1-7). Sydney, Australia: Western Sydney University.
- Edstrom, K. (2017, November 13). The Role of CDIO in Engineering Education Research: Combining Usefulness and Scholarliness. European Journal of Engineering Education.
- Erstad, W. (2017, August 8). Online vs. Traditional Education: What You Need to Know. Retrieved from https://www.rasmussen.edu/student-life/blogs/college-life/online-vs-traditional-education-answer-never-expected/
- Galvis, Á. H. (2018, June 1). Supporting decision-making processes on blended learning in higher education: literature and good practices review. International Journal of Educational Technology in Higher Education, 15(1), 25. Retrieved from https://educationaltechnologyjournal.springeropen.com/articles/
- Gleason, N. W. (Ed.). (2018). Higher Education in the Era of the Fourth Industrial Revolution. Singapore: Springer Nature.
- Graham, R. (2018). The Global State of the Art in Engineering Education. Cambridge, MA, USA: Massachusetts Institute of Technology.
- Ivanov, V. G., Kaybiyaynen, A. A., & Miftakhutdinova, L. T. (2017). Ingenernoye obrazovanie v tsifrovom mire [Engineering Education in Digital World]. Higher Education in Russia, 12(218), 136-143. [in Rus.].
- Jones, L. J. N., & Chew, E. (2015). Blended Learning in Engineering Education: Curriculum Redesign and Development. In S. Tang, & L. Logonnathan (Eds), Taylor’s 7th Teaching and Learning Conference 2014 Proceedings (pp. 441-448). Springer, Singapore.
- Kamp, A. (2016). Engineering Education in the Rapidly Changing World. Rethinking the Vision for Higher Engineering Education. Retrieved from https://repository.tudelft.nl/islandora/object/uuid%3Aae3b30e3-5380-4a07-afb5-dafd30b7b433
- Khalid, M. O. N., Shquier, M. M. A., & Alsmadi, I. (2016). Students performance between classical and online education: a comparative study. International Journal of Continuing Engineering Engineering Education and Life-Long Learning, 26(4), 359-371.
- Kondakov, A. (2017, September 20). Obrazovanie v epokhu chetvertoy promishlennoy revolutsiyi [Education in the era of the fourth industrial revolution]. News of Education, 9(147). [in Rus.]. Retrieved from http://edition.vogazeta.ru/ivo/page-view?obj=14963&pagepos=next
- Miller, G. (2014, July 24). On-Campus vs. Online Degrees: Which One is Better? Retrieved from https://www.worldwidelearn.com/education-articles/online-vs-campus.htm
- Morshed, J. (2017, August 9). 5 Ways to Speed up Digital Transformation in Higher Education. Global VP of Higher Education at Unit4. Retrieved from Microsoft Education website https://educationblog.microsoft.com/2017/08/tips-digital- transformation-higher-ed/
- Ossiannilsson, E., Williams, K., Camilleri, A. F., & Brown, M. (2015). Quality Models in Online and Open Education Around the Globe. State of the Art and Recommendations. Oslo: International Council for Open and Distance Education. Retrieved from: https://www.pedocs.de/volltexte/2015/10879/pdf/Ossiannilsson_et_al_2015_Qualitymodels.pdf
- Reis, J., Amorim, M., Melão, N., & Matos, P. (2018). Digital Transformation: A Literature Review and Guidelines for Future Research. In Á. Rocha, J. Reis, M. Amorim, N. Melão & P. Matos (Eds.), Trends and Advances in Information Systems and Technologies. WorldCIST'18 2018. Advances in Intelligent Systems and Computing, vol 745, (pp. 411- 421). Cham: Springer.
- Richert, A., Shehadeh, M., Willicks, F., & Jeschke, S. (2016). Digital Transformation of Engineering Education - Empirical Insights from Virtual Worlds and Human-Robot-Collaboration. International Journal of Engineering Pedagogy, 6(4), 23-29.
- Schwab, K. (2016). The Fourth Industrial Revolution. Retrieved from: https://luminariaz.files.wordpress.com/2017/11/the-fourth-industrial-revolution-2016-21.pdf
- Sousa, M. J., & Álvaro, R. (2018). Digital learning analytics in higher education. In L. G. Chova, A. L. Martínez, & I. C. Torre (Eds.), 10th International Conference on Education and New Learning Technologies (pp. 787-793). Palma, Spain: IATED.
- van Puffelen, E. A. M. (2017). Designing Blended Engineering Courses. In J. C. Quadrado, J. Bernardino, & J. Rocha (Eds.), Proceedings of the 45th SEFI Annual Conference (pp. 1308 -1312). Azores, Portugal; SEFI.
- Veshneva, I. V., & Singatulin, R. A. (2016). Transformatsiya obrazovaniya: tendentsii, perspective [Transformation of the Education System: Reasons, Tendencies, Perspectives]. Higher Education in Russia, 2(198), 142-147. [in Rus.]
Copyright information
This work is licensed under a Creative Commons Attribution-NonCommercial-NoDerivatives 4.0 International License.
About this article
Publication Date
02 December 2019
Article Doi
eBook ISBN
978-1-80296-072-3
Publisher
Future Academy
Volume
73
Print ISBN (optional)
-
Edition Number
1st Edition
Pages
1-986
Subjects
Communication, education, educational equipment, educational technology, computer-aided learning (CAL), Study skills, learning skills, ICT
Cite this article as:
Chuchalin*, A., Krasina, I., & Kalmanovich, S. (2019). Blended Learning In Engineering Education Based On The Cdio-Fcdi-Ffcd Models. In N. I. Almazova, A. V. Rubtsova, & D. S. Bylieva (Eds.), Professional Сulture of the Specialist of the Future, vol 73. European Proceedings of Social and Behavioural Sciences (pp. 816-826). Future Academy. https://doi.org/10.15405/epsbs.2019.12.86