Abstract
Cognitive control is a set of complex processes supported goal behaviour. A brain basis of cognitive control is studied by special tasks and experimental evidence is integrated in theoretical models. One of the main limitations is that these models are based on data obtained just in individuals with right characteristics of lateral asymmetry. In this study we investigated the functional system of brain structures involved in cognitive control in participants with left eye dominance. 14 left-eyed participants (7 female, mean age = 21.57 ± 1.28) performed Stroop 1, Stroop 2, Stop-signal tasks during fMRI session. Two Stroop tasks included the Russian words “red” and “green” written in red or green font. The subjects asked to press right or left button as a response to presenting of words written in red or green font, respectively (Stroop 1) and to inhibit response to the word “red” and respond only to the word “green” (Stroop 2). In Stop-signal task (with vowel and consonant letters) participants had to press different keys depending on the place of appearance of the green letters and ignore the red letters. The activated areas were revealed (p<0.001) in the contrasts: Stroop 2>Stroop 1 contrast – right precuneus; Stop-signal > Stroop 1 contrast – left occipital pole and left superior occipital gyrus; Stop-signal > Stroop 2 contrast – left occipital pole; Stroop 2>Stop-signal contrast – left precuneus and left cuneus. The results demonstrated the involvement of precuneus in verbal processing and awareness of Stroop stimuli in left-eyed individuals.
Keywords: Cognitive controlleft eye dominancefMR
Introduction
Cognitive control is a set of complex processes supported goal behaviour. A brain basis of cognitive control is studied using special tasks, such as the Stroop task. Based on experiments with the Stroop task Banich supposed a model of cognitive control (Banich, 2009). The “Cascade of Control” model demonstrates a cascade of brain structures activated consistently during interference between meaning and ink of colour words. A consistent involvement of posterior dorsolateral prefrontal cortex (DLPFC), middle DLPFC (mid-DLPFC), and posterior and anterior dorsal anterior cingulate cortex (ACC) is obtained during the Stroop task. Posterior DLPFC establishes the necessary attention settings according to the goal to respond on the colour of the ink and ignore more spontaneous response to the meaning of the word. Further, mid-DLPFC chooses the most suitable representation for the target and selects the relevant information. Next, posterior ACC activate the necessary response. Finally, anterior ACC evaluates the correctness of the response. The activity of this region increases if the error probability grows. In general, the activation level of subsequent regions depends on the previously activated areas. So, if DLPFC spends a lot of resources in choosing the right answer, then anterior cingulate cortex is less activated.
One of the main limitations of Banich’s model is that it is based on data obtained just in individuals with right characteristics of lateral asymmetry. Neuroimaging studies mainly provide evidence of the major role of the right hemisphere. However, the data of brain basis of cognitive control was obtained mostly in individuals with right eye dominance. Therefore, lateralization of this function could be related to different characteristics of eyedness. This reveals a problem of lateral brain organization of cognitive control.
Problem Statement
In this work we focus on cognitive response inhibition as a crucial function of cognitive control. A number of studies reveal a critical role of inferior frontal gyrus and superior frontal gyrus in cognitive inhibition. Inferior frontal gyrus to be critical for the initiation of the stopping process, while superior frontal gyrus is related to top-down control over response inhibition (Aron, 2011; Chambers et al., 2009; Garavan et al., 2006; Swann et al., 2012; Simmonds et al., 2008). Meta-analysis of Swick and co-authors demonstrated that right anterior insula and pre-SMA (presupplementary motor area) were active in the inhibition tasks (Swick et al., 2011). In the Stop-signal task left insula, subcortical structures (thalamus and putamen), posterior cingulate cortex (BA23), right insula, inferior and precentral gyri (BA 9), superior frontal gyrus (medial BA 6), right middle frontal gyrus (BA 9), and right inferior parietal lobule (BA 40) were active. There is an evidence of involvement parietal cortex in inhibition (Kolodny et al., 2017).
Research Questions
To enhance a Banich’s model “Cascade of Control” we used following assumptions. Firstly, we recruited participants with left dominant eye to reveal possible individual features of cognitive control. Secondly, we applied three different tasks. In order to separate inhibition from other possible processes, we used the Stroop task that requires to inhibit some responses and compared it with the classical Stroop task. The Stop-signal with similar (verbal) stimuli is used as additional task.
Purpose of the Study
The aim of this study was to reveal the brain patterns involved in response inhibition in participants with left eye dominance.
Research Methods
Participants
The study included 14 participants (7 female, 7 male, mean age = 21.57 ± 1.28). All the individuals were right-handers and left-eyed. Hand preference was determined according to Annett Hand Preference Questionnaire (Annett, 1970). Eye preference was estimated by neuropsychological probes (Khomskaya, 2006).
Procedure and materials
The main experiment included the Stop-signal task, the classical Stroop task and the Stroop task with additional inhibition. In the Stop-signal task a participants were asked to press different buttons for green vowels and consonants that appeared on the screen. If a red letter appeared a participant was asked to ignore it. The participants were given the following instruction: “You will see green letters. If a vowel appears press the left button of the computer mouse. If a consonant appears press the right button of the mouse. If a red letter appears do not respond”. In the sequence there were 50% of stimuli which required an inhibition of response. Both Stroop tasks included the Russian words “red” and “green” written in red or green font. The subjects had to press the right or the left button as a response to presenting of words written in the red or the green font, respectively in Stroop 1 task. The participants were given the following instruction: “You will see words “green” and “red” written in red or green font. If you see a word written in the green font press the left button of the computer mouse. If you see a word written in the red font press the right button of the mouse”. The same subjects needed to inhibit response to the word “red” and responded only to the word “green” in Stroop 2 task. The participants were given the following instruction: “You will see words “green” and “red” written in red or green font. If you see a word “green” written in the green font press the left button of the computer mouse. If you see a word “green” written in the red font press the right button of the computer mouse. If you see a word “red” do not press buttons of the mouse independently of a font colour”. In the sequence there were 50% of stimuli which required an inhibition of response.
The participants viewed the stimuli which appeared on the screen through a mirror secured inside the tomography. Before each task the participants heard the instruction through a loudspeaker. A block paradigm was used. For the first 15 sec of the session a participant was doing the task, then a 15 sec pause occurred (rest). During the pause a participant viewed a fixation point on a black background. Each session lasted for 2.5 minutes.
MRI data acquisition
The fMRI images were obtained on a Siemens 3T Magnetom Verio scanner. Structural images were acquired with a sagittal T1 MPRAGE protocol using the following parameters: TR = 1470 ms, TE= 1.8 ms, flip angle =9 °, FOV= 250 x 218 mm, 65 slices. Functional images were collected in the axial plane using a T2*-weighted echoplanar image (EPI) gradient-echo pulse sequence covering the whole brain: TR = 2200 ms, TE = 25 ms, flip angle 90°, FOV = 192x192 mm, voxel size = 2*2*2 mm, 65 slices, multiband 5 (CMRR).
Data analysis
The received data were processed using the program SPM12 (p <0.001). During preprocessing images were corrected to exclude artifacts and heterogeneity of magnetic fields. Images were realigned and spatially normalised to coordinates of the Montreal neurological Institute (MNI) standardized space and smoothed with a 8 mm Gaussian filter. The first level analysis was carried out to reveal a contrast “Task > rest” for each participant. The second level analysis included a group comparisons using one-way repeated measures ANOVA with one factor (“task”). This factor included three levels: Stop-signal, Stroop 1 and Stroop 2.
Findings
Stroop 2 > Stroop 1 contrast
Mean group activation was revealed in right precuneus.
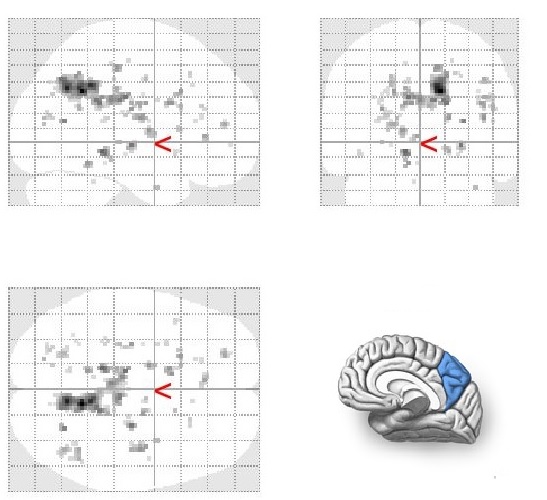
Stop-signal > Stroop 1 contrast
Mean group activation was revealed in left occipital pole and left superior occipital gyrus.
Stop-signal > Stroop 2 contrast
Mean group activation was revealed in left occipital pole.
Stroop 2 > Stop-signal contrast
Mean group activation was revealed in left precuneus and left cuneus.
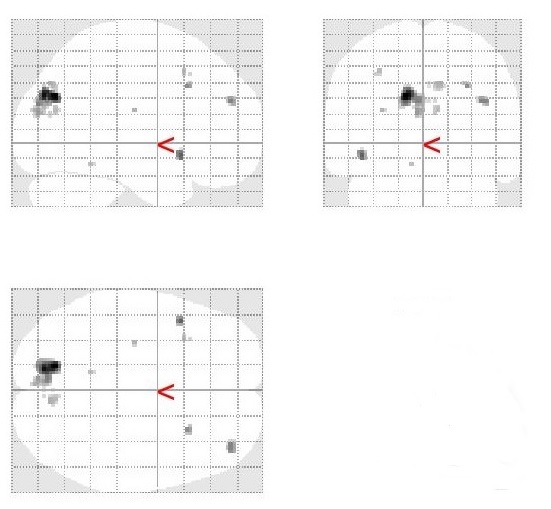
Conclusion
In this study we investigated the functional system of brain structures involved in response inhibition in the participants with left eye dominance. The important role of precuneus in cognitive control in individuals with left-eyedness is demonstrated by the results of the current study. A growing body of studies shows the role of precuneus in episodic memory, verbal politeness, visuospatial abilities and other processes (Fletcher et al., 1995; Farrow et al., 2001; Vogeley et al., 2004; Wenderoth et al., 2005; Oshio et al., 2010). There is an evidence of relationship between precuneus volume and cognitive tasks (including Stroop task) (Garcia et al., 2013) . The study of Garcia and colleagues demonstrated significant (p<0.01 to p<0.0001) correlations between the Stroop scores and right and left precuneus volumes in older adults (mean age 71.5 years). However, there were no correlations in younger participants (mean age 23 years). There is an evidence in literature that precuneus involved in inhibition. van Rooij and coauthors found that subjects with ADHD showed stronger connectivity of superior frontal region with medial frontal, precuneus during successful stops and with temporal areas during failed stops (van Rooij et al., 2015).
A result we achieved is more activated right precuneus in the Stroop 2 task in comparison with the Stroop 1 task. At the same time, the Stroop 1 task did not demonstrate any special activated patterns in comparison with the Stroop 2 task. It cannot be said with certainty however that this result could be related to increasing demand for inhibition of some responses in the Stroop 2 task. The Stroop 2 task performance showed more activated left precuneus and left cuneus in comparison with the Stop-signal and both tasks required inhibition, although the Stroop 2 consists of more complex stimuli (words) than the Stop-signal (letters). Increased activation of precuneus in the Stroop 2 task of the present study is assumed to be relate to more complex verbal processing of the words in comparison with the Stop-signal. Results of the study Kjaer and colleagues supported our conclusion (Kjaer et al, 2001). In this investigation participants recognised three-letter nouns masked by a flow of constantly changing nonwords during PET (positron emission tomography) scanning. Words were presented for 28, 43 or 57 ms. Authors found activated precuneus and dorsolateral prefrontal cortex in enough long periods (43 and 57 ms) and related these regions to awareness of words meaning.
Thus, the results of the present study may complement a “Cascade of Control” model for individuals with left eye dominance. Verbal processing and awareness of Stroop stimuli is present by participation of left precuneus in this group.
Acknowledgments
The research was supported by the Russian Science Foundation (project № 16-18-00066)/
References
- Annett, M. (1970). A сlassification of hand preference by association analysis. British Journal of Psychology, 61(3), 303-321.
- Aron, A.R. (2011). From reactive to proactive and selective control: developing a richer model for stopping inappropriate responses. Biological Psychiatry, 69(12), e55–e68.
- Banich, M.T. (2009). Executive function: The search for an integrated account. Current Directions in Psychological Science, 18(2), 89–94.
- Chambers, C.D., Garavan, H. & Bellgrove, M.A. (2009). Insights into the neural basis of response inhibition from cognitive and clinical neuroscience. Neuroscience & Biobehavioral Reviews, 33(5), 631–646.
- Farrow, T.F., Zheng, Y., Wilkinson, I.D., Spence, S.A., Deakin, J.F., Tarrier, N., Griffiths, P.D. & Woodruff, P.W. (2001). Investigating the functional anatomy of empathy and forgiveness. NeuroReport, 12(11), 2433–2438.
- Fletcher, P.C., Frith, C.D., Baker, S.C., Shallice, T., Frackowiak, R.S.J. & Dolan, R.J. (1995). The Mind's Eye-Precuneus Activation in Memory-Related Imagery. NeuroImage, 2(3), 195–200.
- Garavan, H., Hester, R., Murphy, K., Fassbender, C. & Kelly, C. (2006). Individual differences in the functional neuroanatomy of inhibitory control. Brain Research, 1105(1), 130–142.
- Garcia, A., Luedke, A., Dowds, E., Tam, A., Goel, A. & Fernandez, J. (2013). Precuneus volumes and cognitive tests in older adults. Alzheimer's & Dementia, 9(4), 795.
- Khomskaya, E.D. (2006). Neuropsychology. Spb: Piter.
- Kjaer, T.W., Nowak, M., Kjaer, K.W., Lou, A.R. & Lou, H.C. (2001). Precuneus-prefrontal activity during awareness of visual verbal stimuli. Consciousness and Cognition, 10, 356-65.
- Kolodny, T., Mevorach, C. & Shalev, L. (2017). Isolating response inhibition in the brain: Parietal versus frontal contribution. Cortex. 88, 173–185.
- Oshio, R., Tanaka, S., Sadato, N., Sokabe, M., Hanakawa, T. & Honda, M. (2010). Differential effect of double-pulse TMS applied to dorsal premotor cortex and precuneus during internal operation of visuospatial information. NeuroImage, 49(1), 1108–1115.
- van Rooij, D., Hartman, C. A., Mennes, M., Oosterlaan, J., Franke, B., Rommelse, N., Heslenfeld, D., Faraone, S.V., Buitelaar, J.K. & Hoekstra, P. J. (2015). Altered neural connectivity during response inhibition in adolescents with attention deficit/hyperactivity disorder and their unaffected siblings. NeuroImage. Clinical, 7, 325-335.
- Simmonds, D.J., Pekar, J.J. & Mostofsky, S.H. (2008). Meta-analysis of Go/No-go tasks demonstrating that fMRI activation associated with response inhibition is task-dependent. Neuropsychologia, 46(1), 224–232.
- Swann, N.C., Cai, W., Conner, C.R., Pieters, T.A., Claffey, M.P., George, J.S., Aron, A.R. & Tandon, N. (2012). Roles for the pre-supplementary motor area and the right inferior frontal gyrus in stopping action: electrophysiological responses and functional and structural connectivity. Neuroimage, 59(3), 2860–2870.
- Swick, D., Ashley, V. & Turken, A.U. (2011). Are the neural correlates of stopping and not going identical? Quantitative meta-analysis of two response inhibition tasks. NeuroImage, 56, 1655–1665.
- Vogeley, K., May, M., Ritzl, A., Falkai, P., Zilles, K. & Fink, G.R. (2004). Neural correlates of first-person perspective as one constituent of human self-consciousness. Journal of Cognitive Neuroscience, 16(5), 817–827.
- Wenderoth, N., Debaere, F., Sunaert, S. & Swinnen, S.P. (2005). The role of anterior cingulate cortex and precuneus in the coordination of motor behaviour. European Journal of Neuroscience, 22(1), 235–246.
Copyright information
This work is licensed under a Creative Commons Attribution-NonCommercial-NoDerivatives 4.0 International License.
About this article
Publication Date
23 November 2018
Article Doi
eBook ISBN
978-1-80296-048-8
Publisher
Future Academy
Volume
49
Print ISBN (optional)
-
Edition Number
1st Edition
Pages
1-840
Subjects
Educational psychology, child psychology, developmental psychology, cognitive psychology
Cite this article as:
Marakshina, J., Vartanov, A., & Buldakova, N. (2018). Effect Of Eye Dominance On Cognitive Control. In S. Malykh, & E. Nikulchev (Eds.), Psychology and Education - ICPE 2018, vol 49. European Proceedings of Social and Behavioural Sciences (pp. 402-408). Future Academy. https://doi.org/10.15405/epsbs.2018.11.02.43